
by Elizabeth Gibney
08 March
2017
Nature 543, 164–166
from
Nature Website
Spanish
version
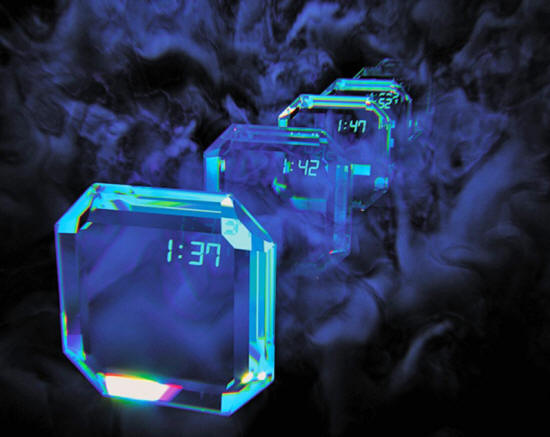
Bizarre forms of matter
called time crystals
were supposed to be
physically impossible.
Now they're not...
Christopher Monroe spends his life poking at atoms with
light.
He arranges them into
rings and chains and then massages them with lasers to explore their
properties and make basic quantum computers.
Last year, he decided
to try something seemingly impossible:
to create a time crystal.
The name sounds
like a prop from Doctor Who, but it has roots in actual
physics.
Time crystals are hypothetical structures that
pulse without requiring any energy - like a ticking clock that
never needs winding. The pattern repeats
in time in much the same way that the atoms of a crystal repeat in
space.
The idea was so
challenging that when Nobel prizewinning physicist Frank Wilczek
proposed the provocative concept
1 in 2012, other researchers quickly proved there was no
way to create time crystals.
But there was a
loophole - and researchers in a separate branch of physics found a
way to exploit the gap.
Monroe, a physicist at the University of
Maryland in College Park, and his team used chains of atoms they had
constructed for other purposes to make a version of a time crystal.
2
"I would say it
sort of fell in our laps," says Monroe.
And a group led by
researchers at Harvard University in Cambridge, Massachusetts,
independently fashioned time crystals out of 'dirty' diamonds.
3
Both versions,
which are published this week in Nature, are
considered time crystals, but not how Wilczek originally
imagined.
"It's less
weird than the first idea, but it's still fricking weird," says
Norman Yao, a physicist at the University of California,
Berkeley, and an author on both papers.
They are also the
first examples of a remarkable type of matter - a collection of
quantum particles that constantly changes, and never reaches a
steady state.
These systems draw
stability from random interactions that would normally disrupt other
kinds of matter.
"This is a new
kind of order, one that was previously thought impossible.
That's
extremely exciting," says Vedika Khemani, part of the Harvard
team and previously part of the group that originally theorized
the existence of the new kind of state.
Experimental
physicists are already plotting how to exploit the traits of these
strange systems in quantum computers and super-sensitive magnetic
sensors.
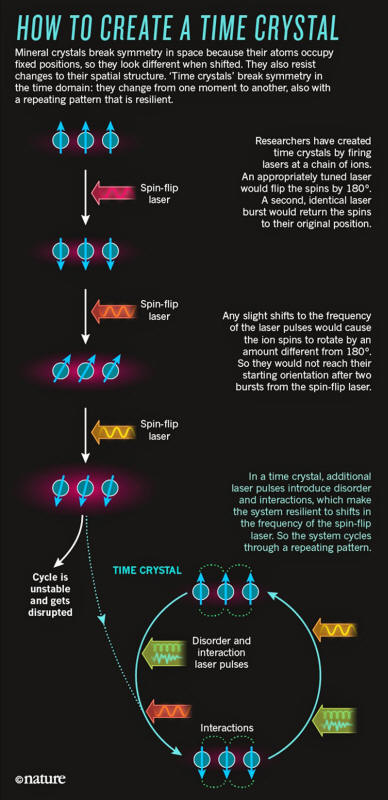
Break time
Wilczek dreamt up
time crystals as a way to break the rules.
The laws of physics
are symmetrical in that they apply equally to all points in space
and time. Yet many systems violate that symmetry. In a magnet,
atomic spins line up rather than pointing in all directions.
In a mineral
crystal, atoms occupy set positions in space, and the crystal does
not look the same if it is shifted slightly.
When a
transformation causes properties to change, physicists call that
symmetry-breaking, and it is everywhere in nature - at the root of
magnetism, superconductivity and even the Higgs mechanism that gives
all particles mass.
In 2012, Wilczek,
now at Stockholm University, wondered why symmetry never broke
spontaneously in time and whether it would be possible to create
something in which it did.
He called it a time
crystal...
Experimentalists imagined a quantum version of this entity
as perhaps a ring of atoms that would rotate endlessly, cycling and
returning to its initial configuration.
Its properties
would be endlessly synchronized in time, just as
atom positions are correlated in a crystal. The system would be
in its lowest energy state, but its movement would require no
external force.
It would, in essence, be a perpetual-motion machine,
although not one that produces usable energy.
"From a first
glance at the idea, one would say this has to be wrong," says
Yao.
Almost by
definition, a system in its lowest energy state does not vary in
time.
If it did, that
would mean it had excess energy to lose, says Norman Yao, and the rotation
would soon halt.
"But Frank
convinced the community that the problem was more subtle than
maybe it seemed to be," he says.
Perpetual motion
was not without precedent in the quantum world: in theory,
superconductors conduct electricity forever (although the flow is
uniform, so they show no variation in time).
These conflicting
issues swam around the head of Haruki Watanabe as he stepped
out of the first oral exam for his PhD at Berkeley.
He had been
presenting work on symmetry breaking in space, and his supervisor
asked him about the wider implications of Wilczek's time crystal.
"I couldn't
answer the question in that exam, but it interested me," says
Watanabe, who doubted such an entity was even feasible.
"I
wondered, 'how can I convince people that it's not possible'?"
Together with
physicist Masaki Oshikawa at the University of Tokyo,
Watanabe began trying to prove his intuitive answer in a
mathematically rigorous way.
By phrasing the
problem in terms of correlations in space and time between distant
parts of the system, the pair derived a theorem in 2015 showing that
time crystals were impossible to create for any system in its
lowest-energy state.
4
The researchers
also verified that time crystals were impossible for any system in
equilibrium - one that has reached a steady state of any energy.
To the physics
community, the case was clear cut.
"That seemed to
be a no-go," says Monroe.
But the proof left
a loophole.
It did not rule out
time crystals in systems that have not yet settled into a steady
state and are out of equilibrium. Around the world, theorists began
thinking about ways to create alternative versions of time crystals.
Particle soup
When the
breakthrough came, it arrived from an unlikely corner of physics,
where researchers weren't thinking about time crystals at all.
Shivaji Sondhi,
a theoretical physicist at Princeton University, New Jersey, and his
colleagues were looking at what happened when certain isolated
quantum systems, made of soups of interacting particles, are
repeatedly given a kick.
Textbook physics
says that the systems should heat up and descend into chaos.
But in 2015,
Sondhi's team predicted that under certain conditions, they would
instead club together to form a phase of matter that doesn't exist
in equilibrium - a system of particles that would show subtle
correlations never seen before - and that would repeat a pattern in
time. 5
That proposal
caught the attention of Chetan Nayak, one of Wilczek's former
students, now at the University of California, Santa Barbara, and at
Microsoft's nearby Station Q. Nayak and his colleagues soon realized
that this strange form of out-of-equilibrium matter would also be a
type of time crystal.
6
But not Wilczek's
kind: it would not be in its lowest energy state, and it would
require a regular kick to pulse.
But it would gain a steady rhythm
that doesn't match that of the instigating kick, and that means it
would break time symmetry.
"It's like
playing with a jump rope, and somehow our arm goes around twice
but the rope only goes around once," says Yao.
This is a weaker
kind of symmetry breaking than Wilczek imagined: in his, the rope
would oscillate all by itself.
When Monroe heard
about this proposed system, he initially didn't understand it.
"The more I
read about it, the more intrigued I became," he says.
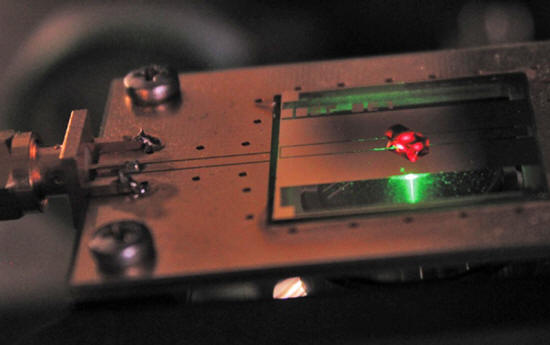
Illumination with green light
reveals a time crystal formed
in a network of electron spins (red)
within the defects of a diamond.
Last year, he
set about trying to form his atoms into a time crystal.
The recipe was
incredibly complex, but just three ingredients were essential:
-
a
force repeatedly disturbing the particles
-
a way to make the
atoms interact with each other
-
an element of random
disorder
The combination
of these, Monroe says, ensures that particles are limited in how
much energy they can absorb, allowing them to maintain a steady,
ordered state.
In his
experiment, this meant repeatedly firing alternating lasers at a
chain of ten ytterbium ions: the first laser flips their spins
and the second makes the spins interact with each other in
random ways.
That
combination caused the atomic spins to oscillate, but at twice
the period they were being flipped.
More than that,
the researchers found that even if they started to flip the
system in an imperfect way, such as by slightly changing the
frequency of the kicks, the oscillation remained the same.
"The system
still locked at a very stable frequency," says Monroe.
Spatial
crystals are similarly resistant to any attempt to nudge their
atoms from their set spacing, he says.
"This time
crystal has the same thing."
At Harvard,
physicist Mikhail Lukin tried to do something similar,
but in a very different system - a 3D chunk of diamond.
The mineral was
riddled with around 1 million defects, each harboring a spin.
And the diamond's impurities provided a natural disorder.
When Lukin and
his team used microwave pulses to flip the spins, they saw the
system respond at a fraction of the frequency with which it was
being disturbed.
Physicists
agree that the two systems spontaneously break a kind of time
symmetry and therefore mathematically fulfill the time-crystal
criteria.
But there is
some debate about whether to call them time crystals.
"This is an
intriguing development, but to some extent it's an abuse of
the term," says Oshikawa.
Yao says that
the new systems are time crystals, but that the definition needs
to be narrowed to avoid including phenomena that are already
well understood and not nearly so interesting for quantum
physicists.
But Monroe and
Lukin's creations are exciting for different reasons, too, says
Yao.
They seem to be
the first, and perhaps simplest, examples of a host of new
phases that exist in relatively unexplored out-of-equilibrium
states, he says. They could also have several practical
applications.
One could be
quantum simulation systems that work at high temperatures.
Physicists
often use entangled quantum particles at nanokelvin
temperatures, close to absolute zero, to simulate complex
behaviors of materials that cannot not be modeled on a classical
computer.
Time crystals
represent a stable quantum system that exists way above these
temperatures - in the case of Lukin's diamond, at room
temperature - potentially opening the door to quantum
simulations without cryogenics.
Time crystals
could also find use in super-precise sensors, says Lukin. His
lab already
uses diamond defects to detect tiny changes in temperature
and magnetic fields.
But the
approach has limits, because if too many defects are packed in a
small space, their interactions destroy their fragile quantum
states.
In a time
crystal, however, the interactions serve to stabilize, rather
than disrupt, so Lukin could harness millions of defects
together to produce a strong signal - one that is able to
efficiently probe living cells and atom-thick materials.
The same
principle of stability from interactions could apply more widely
in quantum computing, says Yao.
Quantum
computers show huge promise, but have long struggled with the
opposing challenges of protecting the fragile quantum bits that
perform calculations, yet keeping them accessible for encoding
and reading out information.
"You can
ask yourself in the future whether one could find phases
where interactions stabilize these quantum bits," says Yao.
The story of
time crystals is a beautiful example of how progress often
happens when different strands of thought come together, says
Roderich Moessner, director of the Max Planck Institute for
the Physics of Complex Systems in Dresden, Germany.
And it may be,
he says, that this particular recipe proves to be just one of
many ways to cook up a time crystal.
References
-
Wilczek, F.
Phys. Rev. Lett.
109, 160401 (2012)
-
Quantum Time Crystals
-
Zhang, Z.
et al. Nature
543,
217–220 (2017)
-
Observation of a discrete time
crystal
-
Choi, S.
et al. Nature
543,
221–225 (2017)
-
Observation of discrete
time-crystalline order in a disordered dipolar many-body
system
-
Watanabe, H.
& Oshikawa, M.
Phys. Rev. Lett.
114, 251603 (2015)
-
Absence of Quantum Time Crystals
-
Khemani, V.,
Lazarides, A.,
Moessner, R.
& Sondhi, S. L.
Phys. Rev. Lett.
116, 250401 (2016)
-
Phase Structure of Driven Quantum
Systems
-
Else, D. V.,
Bauer, B.
& Nayak, C.
Phys. Rev. Lett.
117, 090402 (2016)
-
Floquet Time Crystals
|