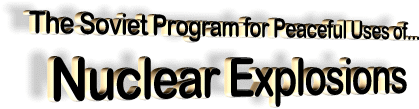
by Milo D. Nordyke
1998
Science & Global Security, 1998,
Volume 7, pp. 1-117
from
PrincetonUniversity Website
Milo D. Nordyke
Formerly Director,
Lawrence Livermore National Laboratory, Center for
Studies of Security, Energy and Arms Control, Livermore,
California. |
This paper presents an historical review
of the Soviet program to study and utilize industrial applications
of peaceful nuclear explosions (PNEs) in the Soviet Union over the
period 1965 to 1988.
This was a very active program that
carried out 122 nuclear explosions to study some 13 applications.
In
all, 128 nuclear explosives with yields ranging from 0.01 to 140 kt
(kiloton)
were used, with the vast majority being between 2 and 20 kt. Over
half of these explosions were used for implementation of two
industrial applications: the creation of underground cavities in
salt for the storage of gas condensate and deep seismic sounding of
the Earth's crust and upper mantle.
Other applications studied with nuclear
explosions included oil and gas stimulation, closure of runaway gas
wells, disposal of toxic chemical wastes, block cave mining of
underground minerals, production of
transplutonic elements, and the
excavation of water reservoirs and canals.
In terms of the number of applications explored with field
experiments, the Soviet PNE program was an order of magnitude larger
than the U.S. "Plowshare" program carried out in the 1960s and early
1970s; the U.S. utilized no explosions on an industrial basis and
fired only three industrial field experiments.
The Soviet program also included the
development of low-fission excavation explosives and specially
designed hydrocarbon explosives, which mirrored the device
development program in the U.S. Plowshare program. The Soviet PNE
program was terminated in 1989 as part of the unilateral Soviet
moratorium on nuclear weapons testing adopted by President
Gorbachev.
The Comprehensive Test Ban Treaty
recently signed by Russia and the United States includes a ban on
all nuclear explosions, including those for peaceful uses.
EARLY HISTORY
The concept of utilizing the weapons of
war to serve the peaceful pursuits of mankind is as old as
civilization itself.
Perhaps the most famous reference to
this basic desire is recorded in the Book of Micah where the great
prophet Isaiah called upon his people,
"to turn your spears into pitchforks
and your swords into plowshares."
As the scientists at Los Alamos worked
on developing the world's first atomic bomb, thoughts of how this
tremendous new source of
energy could be used for peaceful purposes generally focused on
using the thermal energy generated by the slow fission of uranium in
a reactor, such as those being used to produce plutonium, to drive
electric power stations.
However, being scientists in a new, exciting field, it was
impossible to avoid letting their minds wander from the task at hand
to other scientific or nonmilitary uses for the bombs themselves.
During the
Manhattan Project, Otto Frisch, one of the pioneers in
the development of the nuclear fission process in the 1930s, first
suggested using an atomic explosion as a source for a large
quantities of neutrons that could be used in scientific experiments
designed to expand the understanding of nuclear physics.
After the war was over, many grandiose
ideas appeared in the popular press on how this new source of energy
should be harnessed to serve mankind.
Not to be left out of the growing enthusiasm for peaceful uses of
atomic energy, the Soviet Union added their visions to the public
record. In November 1949, shortly after the test of their first
nuclear device on September 23, 1949, Andrei Vishinsky, the Soviet
representative to the U.N., delivered a statement justifying its
efforts to develop its own nuclear weapons capability.
In poetic but somewhat overblown
rhetoric, he said:
The Soviet Union did not use atomic
energy for the purpose of accumulating stockpiles of atomic
bombs, ...it was using atomic energy for purposes of its own
domestic economy: blowing up mountains, changing the course of
rivers, irrigating deserts, charting new paths of life in
regions untrodden by human foot.1
A few years later, a Russian engineer,
Professor G. I. Pokrovskiy wrote:
Progressive science claims that it
is possible to utilize the noble force of the explosions builder
for peaceful purposes.... With the help of directional
explosions one can straighten out the beds of large
rivers...construct gigantic dams...cut canals.... Indeed, the
perspectives disclosed due to the new atomic energy are
unlimited.2
However, very few of the articles
written in the late 1940s and early 1950s had concrete ideas on how
the explosive force of the bombs themselves could be used for
scientific purposes or to transform the landscape and alter the
character of geological formations deep under the earth.
One of the first was written by Fred
Reines, a young physicist who had come to Los Alamos in 1944 to
work on the nuclear weapons program.
In June of 1950, he wrote a short
article for the Bulletin of the Atomic Scientists examining the
possibilities of using atomic explosives for a few large-scale,
earth-moving applications such as, making canals, mining, breaking
up icebergs, and melting the polar icecap.
In general, his outlook
was rather pessimistic, concluding that,
"such uses appear at best to be
extremely limited in scope, owing to the radioactivity hazard
associated with atomic explosions."3
With the development of thermonuclear
devices, new ideas began to ferment in the minds of the
bomb-designers.
Thermonuclear devices still required a
small fission trigger, but since the thermonuclear fuel consisted of
relatively cheap deuterium and lithium and produced almost no
long-lived radioactive byproducts, they offered the possibility of
an order-of-magnitude decrease in both the cost of an explosive and
the amount of radioactivity associated with a given total yield.
The detonation by the Soviet Union of
their first thermonuclear explosion on August 12, 1953, led
President Eisenhower to the determination that he needed to take the
initiative in dealing with the political aspects of the nuclear arms
race.
Toward this end on December 8,1953, President
Eisenhower
delivered his now-famous Atoms for Peace speech at the U.N. calling
for,
...more than the mere reduction or elimination of atomic
materials for military purposes.
It is not enough to take this weapon out of the hands of their
soldiers. It must be put into the hands of those who will know how
to strip its military casing and adapt it to the arts of
peace...this greatest of destructive forces can be developed into a
great boon for the benefit of all mankind....
Who can doubt, if the entire body of the world's scientists and
engineers had adequate amounts of fissionable material with which to
test and develop their ideas, that this capability would rapidly be
transformed into universal, efficient, and economic usage.
This dramatic and stirring call to the world community to begin the
process of applying this powerful new source of energy to the
peaceful uses of mankind served as a powerful stimulant within the
nuclear physics community and nuclear power industry.
Following up
on Eisenhower's Atoms for Peace speech, in early 1954 the U.S.
proposed that the U.N. sponsor a Conference on the Peaceful Uses of
Atomic Energy.
The first of four such conferences was
ultimately held in Geneva, Switzerland, in August of 1955. It was
the largest scientific meeting in the world held up to that time,
with over 2,500 participants in attendance; more than 1,000
technical papers were presented. For many Soviet scientists, it was
their first opportunity to attend a scientific meeting outside the
Soviet Union and to meet their colleagues from the West.4
Although no papers were presented at the Geneva Conference on the
peaceful uses of nuclear explosions, the general enthusiasm for the
integration of peaceful uses of nuclear energy into the fabric of
society and the declassification of a broad spectrum of information
about the attributes and effects of nuclear fission processes gave
rise to an increasing interest in such ideas, particularly within
the nuclear weapons community.
Fired by this enthusiasm, in the spring of 1956, a French scientist
named Camille Rougeron wrote a monograph conjuring up images of a
wide variety of applications for such explosions - building dams,
changing the course of rivers, melting glaciers, breaking up ice
jams, changing the climate, constructing underground power plants
driven by the heat of thermonuclear explosions, and breaking rock
for mining.5
Rougeron's "dreams" added little in the
way of quantitative analysis of such applications, but they did
serve to raise the expectations of the general public for some
peaceful benefit from the nuclear tests being fired in the Pacific
and at the Nevada Test Site.
At about the same time, the Soviet engineer G. I. Pokrovskiy
again wrote of his vision of using compact, powerful, low-cost
nuclear explosives for removing overburden from valuable ore
deposits or excavating canals:
With the data now available, we can
say that radioactive contamination in a nuclear explosion should
not be considered an insurmountable obstacle to the use of such
explosives in mining and construction. On the basis of the many
advantages of nuclear explosions, we conclude that the time is
ripe to begin actual experiments in this field.6
THE U.S. PLOWSHARE PROGRAM
In spite of Pokrovskiy's enthusiasm,
little of substance was done over the next ten years in the Soviet
Union to further explore his vision.
In the U.S., however, the AEC formally
established a program for nonmilitary uses of nuclear explosions in
the summer of 1957. Named
Project Plowshare, the emphasis in the
first year was placed on studies aimed at further fleshing out the
physics and engineering aspects of using underground nuclear
explosions for power generation, on beginning nuclear design work on
very low fission explosives especially designed for nuclear
excavation, and on finding a suitable site for a near-term
demonstration of this new technology.
In the fall of 1957, the AEC carried out the world's first
underground nuclear explosion, the Rainier event, a 1.7-kt test
emplaced in a tunnel 274 m below a mesa at the Nevada Test Site (NTS).
Although it was a weapons test, the
purpose was primarily to document the effects of an underground
nuclear explosion. Results from the Rainier test gave the Plowshare
project a tremendous boost of enthusiasm and confidence that a
variety of peaceful uses for nuclear explosions were possible and
could be implemented safely.
Before Rainier, all the ideas for
peaceful uses were based on theoretical conjecture about the
interaction of nuclear explosions with their surroundings. Now the
scientists had actually fired an underground explosion, and
everything happened about as expected. Thus, Rainier validated many
of the Plowshare concepts that had been only sketchy ideas in
scientists' minds and gave new confidence that these ideas would
work.
In an angry press conference following the presentation of results
by Gerry Johnson at the Second International Conference on the
Peaceful Uses of Atomic Energy in Geneva in September 1958, Vasiliy
Emelyanov, the Chief Soviet Delegate to the Conference, attacked the
U.S. Plowshare Program as a subterfuge for continued nuclear weapons
testing and scoffed at Plowshare's potential.
He disavowed past statements by Soviet
scientists, engineers, and politicians expressing interest in such
applications and condemned such explosions,
"as a 'cover' to evade suspension of
bomb tests" which "do not reach practical ends, but only
political ends."7
Undeterred by Emelyanov's negative
comments, the U.S. proceeded with development of the Plowshare
Program.
The first field experiment sponsored by
the Plowshare Program was Project Gnome in 1961, a 3.1-kt explosion
at a depth of 367 m in a bedded salt formation near Carlsbad, New
Mexico. The general purpose was to study the effects of a nuclear
explosion in salt, a unique medium that is able to sustain extremely
large cavities without collapse, with a view to the use of such
cavities for a variety of peaceful purposes.
At that time, the possible use of such
cavities for decoupling the seismic signal from clandestine nuclear
weapons tests was also a controversial arms control issue in the
test ban negotiations that were on-going in Geneva.
The U.S. invited observers from all the
U.N. countries to view the Gnome explosion, but the Soviet Union,
consistent with their Geneva position, refused to participate. The
Gnome explosion was a technical success, providing much data on
scientific experiments and the effects of nuclear explosions in
salt. It also resulted in a public relations disaster when a leak
developed in the tunnel stemming, and a cloud of radioactive gases
escaped shortly after the explosion.8
Over the next 15 years, the U.S. Plowshare Program studied a variety
of possible applications for peaceful nuclear explosions.
Table 1
provides a list of the field experiments sponsored by the U.S.
Plowshare Program. In the early years, primary emphasis was placed
on the development of technologies for nuclear excavation, the
application that appeared most economically attractive and
technically straightforward.
Following an abortive plan to excavate a
demonstration harbor in northern Alaska that was abandoned in 1962,
almost all excavation research was directed at the technical
challenges of using nuclear explosions to excavate a new sea-level
canal through the Central American Isthmus to replace the Panama
Canal.
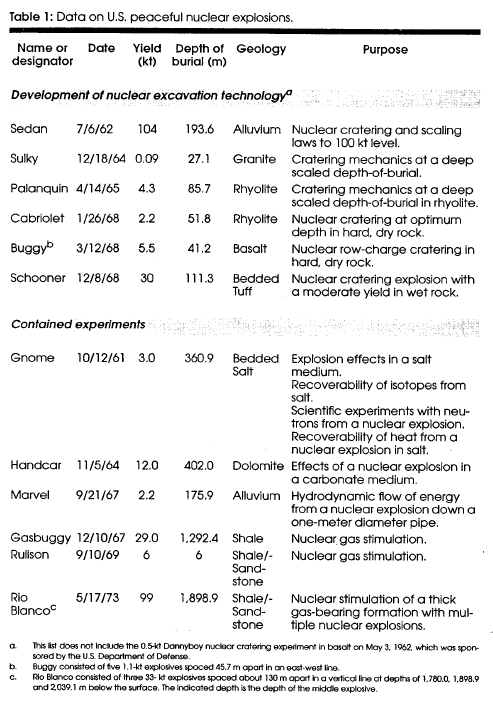
This effort was in direct support of the
Atlantic-Pacific Interoceanic Canal Study Commission (APICSC),
appointed by President Lyndon Johnson in 1965, and continued until
the Commission delivered its final report in December, 1970.
As part of this effort, Plowshare carried out six nuclear cratering
experiments between 1961 and 1968 with yields ranging from 0.1 to
100 kt. All were conducted at the NTS. In an effort to ameliorate
the primary hazard from nuclear excavation - the radioactivity
released to the atmosphere - a major part of this program was the
development of special ultra-low-fission nuclear explosives designed
for excavation applications.
This special excavation explosive
development program required nine tests at NTS from 1963 to 1970 and
resulted in an explosive design with various yields with a fission
yield so low that, when used in a cratering application, the fission
products released to the atmosphere by each explosive would be less
than that created by a 20-ton fission explosion.
With the conclusion of the APICSC study
and the rising public sensitivity to environmental contamination,
the nuclear excavation portion of the U.S. Plowshare Program was
phased out in the early 1970s.
Starting with the
Gnome experiment in 1961, the Plowshare Program
provided continued support for scientific experiments, primarily as
additions to weapons tests, utilizing the extremely high fluxes of
neutrons available within and near nuclear explosions to conduct
experiments impossible with other neutron sources. In the early
1960s, a major program effort was undertaken to look at the
possibility of using these high neutron fluxes to produce heavy transplutonic elements well beyond the end of the Periodic Table.
The ultimate goal was the use of
multiple neutron captures to reach the predicted "island of
stability" at element 114.
Between 1962 and 1969, Plowshare
supported the design and fielding of five dedicated experiments and
"add-ons" to some ten weapons tests at NTS in a futile attempt to
reach this elusive goal. However, very large quantities of some
heavy elements were produced, of which only trace fractions were
recovered from the melt zone.
The last isotope production experiment
on the Hutch event produced an estimated neutron flux on the target
material of 40 mols/cm2 and produced over 1017
atoms of Fm-257 - 104 more heavy elements than any
previous experiment - and over 1020 atoms of Cm-250. More
than 1010 atoms of Fm-157 were recovered, 100 times more
than had been produced by any other method to that date.
In addition to these scientific programs, the Plowshare Program also
carried out two experiments in the mid-1960s to learn more about the
effects of nuclear explosions. The Handcar experiment in 1964 was a
12-kt contained explosion fired at NTS in a dolomite formation,
consisting of a mixture of calcium and magnesium carbonate.
Prior to the Handcar test, explosions in
rock containing a high carbonate content had been avoided because of
concerns regarding containment of the large quantities of
non-condensable CO and CO2 gas produced by a nuclear
explosion in such a medium. Results from the Handcar explosion
demonstrated that such nuclear explosions could be carried out with
complete containment in such a medium.9
The second experiment was the Marvel test, also at NTS, in 1967 to
study the propagation of a shock wave from a nuclear explosion along
a horizontal, air-filled tunnel, one meter in diameter and 122 m
long, which was immediately adjacent to the nuclear explosion. The
primary purpose was to develop techniques for understanding the
propagation of energy in a non-spherical geometry.10
Among the industrial applications utilizing a completely contained
explosion, the most intense studies were directed at the stimulation
of gas production from low-permeability gas reservoirs, the recovery
of oil from the vast oil shale deposits in Colorado, the breakage of
copper ore preparatory to in-situ leaching, and the creation of
cavities for the storage of oil and natural gas.
Only gas stimulation found sufficient industrial support to proceed
to actual field experiments.11
From 1967 to 1973, three joint
industry-government experiments were carried out in very low
permeability gas fields (see table 1). In all cases, the explosions
were carried out without incident, and significant increases were
realized in the production of gas over that experienced from nearby
conventional wells.12
The most significant radiological concern was the incorporation of
tritium produced by the nuclear explosive into the gas produced from
the stimulated region. To reduce emplacement costs and tritium
levels to the lowest possible levels, the Plowshare Program
developed a special nuclear explosive less than 200 mm (7.8 in) in
diameter that produced an extremely small amount of tritium (<0.2
g), primarily by neutrons in the medium surrounding the explosive.
Three of these special explosives were used in the same hole for the
Rio Blanco event, one above the other spaced about 130 m apart.
Although projected public radiation exposures from commercial use of
stimulated gas had been reduced to less than one percent of
background,13 it became clear in the early 1970s that public
acceptance within the U.S. of any product containing radioactivity,
no matter how minimal, was difficult if not impossible. In addition,
the economic viability of nuclear gas stimulation would require the
stimulation of hundreds of wells over several decades, a prospect
that proved daunting to potential industrial sponsors in light of
growing public concerns about environmental quality.
Following completion of the post-shot
gas-production testing of Rio Blanco in December, 1974, the gas
stimulation program, together with the studies of other potential
Plowshare applications, was rapidly phased down, and the U.S.
Plowshare Program was terminated in 1977.
In summary, during its 20-year life, the U.S. Plowshare Program
carried out twelve field experiments, six nuclear cratering events,
and six contained explosions. Only four Plowshare events were
conducted off the Nevada Test Site, one to better understand the
effects of a nuclear explosion in salt and three for nuclear gas
stimulation.
In addition to these experiments, the program also fully funded 16
device development tests at NTS. Five of these tests, together with
eight add-ons to weapons tests, were in pursuit of the goal of
developing super-heavy transplutonic elements.
Nine were for the purpose of developing
an ultra-low-fission thermonuclear explosive for use in nuclear
excavation projects, and one each were for the development of
special emplacement techniques and for a small-diameter,
ultra-low-tritium-producing explosive for hydrocarbon applications
such as Rio Blanco.
THE SOVIET PROGRAM FOR THE
USE OF NUCLEAR EXPLOSIONS IN THE
NATIONAL ECONOMY
An Historical
Perspective
The Soviet Union did not immediately follow the U.S. lead in 1958 in
establishing a program to investigate the peaceful uses of nuclear
explosions.
Presumably, its political position in
support of a comprehensive nuclear test ban, which would have banned
or strongly discouraged such explosions, forestalled any efforts to
establish such a program until the mid-1960s.
At some point during this time frame, the Soviet Union formally
established "Program No. 7 - Nuclear Explosions for the National
Economy."
Alexander D. Zakharenkov, a chief weapons designer
at the Chelyabinsk-70 nuclear weapons laboratory was named to head
the program, and Oleg L. Kedrovskiy to be the chief scientist.
Initially, the Soviet program was
focused on two applications, nuclear excavation and oil stimulation,
as the U.S. Program had been. However, interest in other
applications quickly developed, and within five years the Soviet
program was actively exploring six or seven applications involving
participation by some ten different Ministries.14
One of the first steps in developing such a program was initiated by
Efrim P. Slavskiy, former Minister of the Medium Machine
Building Ministry (the ministry responsible for the entire Soviet
nuclear weapons program).15
He was undoubtedly aware of the
activities of the U.S. Plowshare program and was reported to be an
avid supporter of using nuclear explosions for industrial purposes.
On his directive on January 15, 1965, his Ministry sponsored a
large-yield (140 kt) cratering explosion carried out at a depth of
178 m on the edge of the Semipalatinsk Test Site (STS) in northern
Kazakhstan, which formed a large lake. Minister Slavskiy was
reported to have been the first person to have taken a swim in the
crater lake.16
Later in 1965, in cooperation with the Ministry of the Oil Industry,
Program No. 7 began field experiments looking at the possibility of
using nuclear explosions to increase oil production as well as
planning experiments in salt to produce cavities. The nuclear
weapons laboratory at Arzamas-16 (All-Soviet Institute of
Experimental Physics-VNIIEF) near Gorky initially played the major
role in Program No. 7, adapting military explosions to peaceful
applications.
The laboratory at Chelyabinsk-70
(All-Soviet Institute of Technical Physics-VNIITF) soon became
involved and, over the years, became the most active participant in
the program, particularly in the design of special nuclear
explosives for particular applications.17 Models of the
special nuclear explosives can be seen in the Chelyabinsk-70 Nuclear
Weapons Museum in Snizhinsk.
In November of 1965, a conference was held in the Soviet Union to
consider possible industrial and scientific uses for nuclear
explosions. The meeting included the leading scientists and weapons
designers in the Soviet nuclear weapons program, including Andrei
Sakharov. The scientists evinced great interest in such a program,
including the development of special explosives to facilitate the
fielding of nuclear explosives in unique industrial situations and
to reduce the radioactivity produced by such explosions.
The ideas discussed ranged from
scientific experiments and industrial applications utilizing the
unique physical and electromagnetic properties of nuclear explosions
to control asteroids and power rockets in deep space.18
In the middle of 1966, a crisis in the gas industry suddenly offered
an opportunity for a new application for peaceful nuclear
explosions, the extinguishing of runaway gas wells. Successfully
closing several such wells in 1966 and 1967 gave growing confidence
to the leaders of the program, and they began to think about a broad
spectrum of new applications.
In the spring of 1969, the Soviet Union approached the U.S. with a
proposal to engage in a series of bilateral discussions on peaceful
nuclear explosions. The first of a series of four such meetings was
held in Vienna, Austria, on April 14-16, 1969. Subsequent meetings
were held in Moscow (February 12-17, 1970), Washington (July 12-23,
1971), and Vienna (January 15-17, 1975).19
In the course of these meetings with
scientists from the U.S. Plowshare Program, Soviet scientists
cautiously unveiled some of the technical details of the first few
PNE experiments as well as general plans for several applications
they were developing. In the early 1970s, the Soviet Union also
provided information on the scope and technical results of some of
their program activities through a series of Panel Meetings on
Peaceful Nuclear Explosions at the International Atomic
Energy Agency (IAEA) in Vienna, Austria.20
A few articles appeared in the Russian
press in the early seventies describing the general purposes of the
Soviet PNE Program, but no specifics on locations or results were
given. Several articles were written in the U.S. in the
mid-seventies and early eighties describing what was known at that
time about the program from these meetings and from seismic signals
coming out of the Soviet Union.21,22,23
However, since the mid-seventies, little
technical information about the program was made available until the
advent of "glas-nost" in the late 1980s. Since that time, there have
been news reports and commentaries by environmental groups about the
Soviet PNE Program in the newly "opened" press, but there has been
little authoritative information.
However, a recently published book by
the Khlopina Radium Institute 24 and several
articles in the Information Bulletin of Center for Public
Information on Atomic Energy, published by the Russian Atomic Energy
Ministry, have provided a good look into the overall scope,
technical details, and industrial results of this program.
Overview of
the Soviet PNE Program
Since its inception in 1965, the Soviet PNE program carried out 122
explosions involving approximately 128 explosives to study some 13
potential uses.25
Five applications were put into
industrial use (e.g., cavities for storing gas condensate and deep
seismic sounding of the earth's mantle). Table 2 summarizes these
explosions in terms of their general purpose. In all, PNE explosions
were carried out at 115 sites located throughout the former Soviet
Union. Two sites were re-entered for subsequent explosions,
utilizing the cavities produced in salt by earlier explosions.
Tests carried out at the test sites for
the development of special nuclear explosives or emplacement
techniques for PNEs are not included in the above totals (see
Appendix C).
The Soviet program came to an end with the adoption by the Soviet
Union of a unilateral moratorium on the testing of nuclear weapons
at Soviet Test Sites in 1989.
Although it primarily was designed to
support the Soviet Union's call for a world-wide ban on all nuclear
weapons tests, the Soviet Union also applied the moratorium to
nuclear explosions for peaceful purposes.
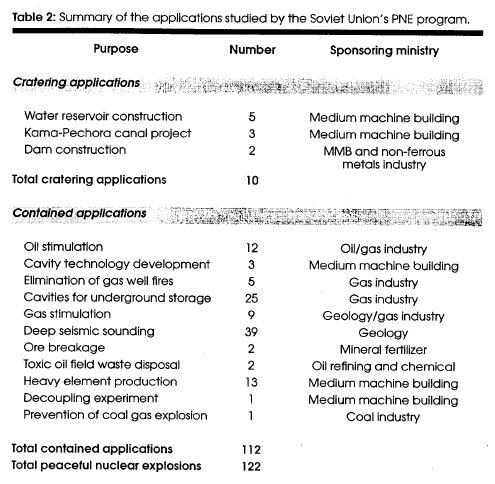
Data on the 122 explosions of the Soviet
"Program for the Utilization of Nuclear Explosions in the National
Economy" are presented in "Appendix A" far below.
The numbers of the explosions are given
in chronological order, along with their names, dates and times,
seismic locations, magnitudes, and general geographic locations.
Actual times and locations are also provided, when available. Figure
1 is a map of the former Soviet Union showing the geographic
locations of the 122 PNE sites.
"Appendix B" lists the 122 explosions
grouped chronologically within some 13 different applications. The
information provided includes the yield, depth of burial, geological
medium surrounding the emplacement point, general comments about the
explosions, and the sponsoring ministries.
The remainder of this
report discusses the activities for each application from an
historical perspective.
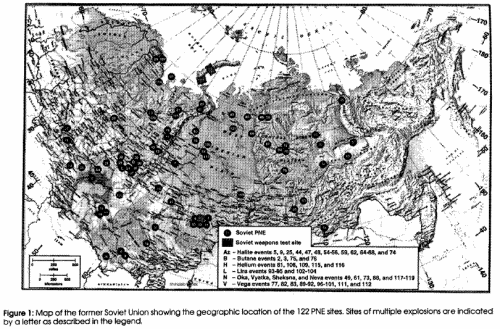
The Nuclear
Excavation Program
Water Reservoir Construction
One of the first applications considered for peaceful nuclear
explosions in the Soviet Union was the development of water
reservoirs to improve agriculture in such vast arid areas of Siberia
as the Semipalatinsk, Kustanay, Tselino-grad, Pavlodar, and Gur'ev
regions.
Many rivers and streams in these regions flow during times
of high rainfall but are dry the remainder of the year.
The suggested application envisaged creating nuclear craters within
or adjacent to these intermittent streams with volumes of 3 to 5
million cubic meters of storage capability.
Chagan
The first experiment in the Soviet PNE Program on January 15, 1965,
was directed at the general goal of obtaining data on the use of
nuclear explosives for cratering purposes as well as the specific
purpose of demonstrating the usefulness of nuclear explosives in
creating water storage reservoirs (see section A.l of Appendix B).
This experiment utilized a 140-kt explosive placed 178 m deep in
Hole 1004 on the edge of the Semipalatinsk Test Site (STS) in
Kazakhstan.
The site was chosen to be in the dry bed
of the Chagan River so that the crater lip would form a dam in the
river during its period of high flow in the spring.
The crater formed by the Chagan explosion had a diameter of 408 m
and a depth of 100 m, remarkably similar to the Sedan crater at NTS.
A major lake was quickly formed behind the 20-35 m high upraised
lip.
Shortly after the explosion, earthmoving
equipment was used to cut a channel through the lip so that water
from the river could enter the crater. Spring melt soon filled the
crater with 6.4 million m3 of water, and the reservoir behind the
crater was filled with 10 million m3 of water. Subsidence of the
crater slopes subsequently reduced the crater storage capacity by
about 25 percent.
A few years later, a water-control
structure was built on the left bank of the river to control water
levels in the reservoirs. Both reservoirs exist today in
substantially the same form and are still used to provide water for
cattle in the area (see figure 2).
The crater dimensions for the Chagan crater compared very well with
the 100-kt Sedan crater at NTS, even though the media were quite
different and the scaled depth-of-burial of Chagan was almost 20
percent less than that at Sedan.
Whereas the medium at Sedan was dry
desert alluvium with a moisture content below one percent, the
medium at Chagan was saturated silt-stone with a 12 percent water
content.
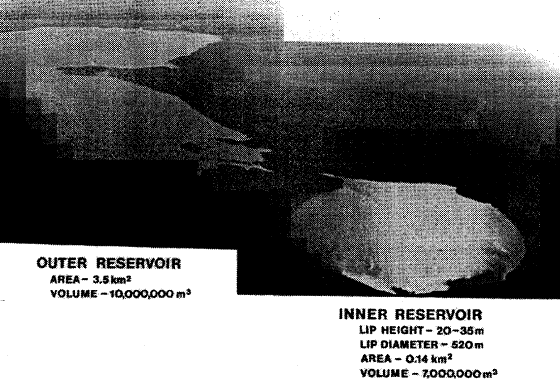
Figure 2:
Photographic
composite of "Chagan" Lake showing the "Inner Reservoir,"
formed by the Chagan
crater, and the "Outer Reservoir, "
formed by the
blockage of the Chagan River by the lip of the crater.
The channel
constructed through the lip into the crater shortly
after the explosion
Is visible in the center of the photograph.
The channel and
control structure to bypass water around the dam
is located to the
left of the crater lip in the left side of the photograph.
The nuclear explosive used for the
Chagan test was reported to be a low-fission design, which had a
pure thermonuclear secondary driven by a fission
primary with a yield of about 57 kt.26
Approximately 20 percent of the
radioactive products of the explosion escaped into the atmosphere,
resulting in dose levels on the lip of the crater of 20-30
µR/hr
several days after the explosion, most of which was from Co60 (5.26
year half-life). Today, the dose level on the lip is reported to be
-2.6 mR/hr.27
Beyond a restricted area 100-150 m from
the lip, the dose rate is at background levels (15-20
µR/hr).28
Radioactivity levels in the lake water in the crater are reported to
be about 300 pCi/liter.29
Radioactivity from the Chagan test was detected over Japan by both
the U.S. and Japan in apparent violation of the 1963 Limited Test
Ban Treaty (LTBT). The U.S. complained to the Soviet Union about
the explosion, interpreting it as an accidental venting of a
high-yield weapons test and asking for an explanation.
The Soviets
responded that the explosion,
"was carried out deep underground. The quantity of radioactive debris that
leaked into the atmosphere was so insignificant that the possibility
of its fallout outside the territorial limits of the Soviet Union
should be excluded."
After several subsequent interactions, the
issue was closed without further explanation.30
Soviet scientists attributed the venting of 20 percent of the
radioactivity in the Chagan test to the fact that the scaled
depth-of-burial of the charge, 42 m/kt1/3.4, was somewhat
less than optimum.
The fact that the rock surrounding the
explosion was water-saturated almost certainly contributed to the
relatively high escape fraction.
Sarv-Uzen
Later that same year on
October 10, 1965, Soviet scientists decided to carry out a second
nuclear cratering experiment at a scaled depth that was thought to
be closer to optimum than the Chagan test.
For this explosion, a 1.1-kt explosive
was emplaced in Hole 1003 at a depth-of-burial of 48 m (scaled
depth-of-burst = 46.7 m/kt11/3-4) in the dry bed of the Sary-Uzen'
stream on the western edge of the Semipalatinsk Test Site. At shot
depth, the geologic medium was a weak siltstone rock, similar to
sandstone. However, it was overlain by about 10 m of clay-like
material.
The explosion produced a crater with an initial diameter
of 107 m and depth of 31 m.
The dimensions of the initial Sary-Uzen' crater compared favorably
with U.S. experience in dry alluvium. However, within three months,
the crater flooded with artesian water from the shallow water table,
resulting in sluffing of the slopes of the crater, which reduced the
depth of the crater to 20 m and increased the diameter to 124 m.
Soviet scientists had pre-emplaced a
high-explosive line-charge under one area of the expected Up, and
this was detonated several minutes after the nuclear explosion. This
line-charge produced a "canal" through the crater lip to allow
stream flow to enter the crater without any personnel re-entering
the crater area.
For the Sary-Uzen' cratering explosion, only 3.5 percent of the
radioactivity produced in the explosion escaped into the atmosphere.
Five days after the test, the dose rate on the lip was 2-3
µR/hr.
Today it is reported to be 50
µR/hr. Beyond the lip area, dose rates
are at background.31
Although the Soviets professed to see a widespread need in arid
regions of the U.S.S.R. for more than 50 water storage reservoirs
with storage capacities in the range of 3-5 million m3,
which would require cratering explosions of 20-50 kt, no further
experimentation or application of the technologies demonstrated in
Chagan and Sary-Uzen' were carried out.
Holes 2-T, 1-T, and 6-T
Several years after the
Chagan and Sary-Uzen' experiments, the Soviet PNE program carried
out three additional experiments that they reported were directed at
the development of water reservoirs in arid locales.
For some years, Soviet scientists had
noted the U.S. experience at NTS, where large-yield nuclear
explosions in alluvial media resulted in large subsidence craters on
the surface above the explosion with essentially no release of
radioactive material.
Such craters result from the collapse of an explosion cavity and all
the material lying above it, so that at the surface above, a large
fraction of the volume of the cavity appears in the form of a
conical subsidence. This can only occur when the medium above the
cavity is of such a nature that it doesn't "bulk" 32 when
it collapses into the explosion cavity. The deep alluvial deposits
at NTS are ideally suited for the formation of subsidence craters.
The resulting crater has no upraised lip, and the diameter and depth
can vary greatly, even in the same media.
The Soviets believed such structures might be useful for water
reservoirs. Because the geological media at their test sites at
Semipalatinsk and Novaya Zemlya were all igneous or sedimentary
rocks, they had not experienced this phenomenon. They decided to
gain some direct experience at a remote site on the Mangyshlak
Plateau mid-way between the Aral and the Caspian Seas where they had
found a weak, high-porosity sedimentary formation at the appropriate
depth.
Three experiments described as being for the purpose of studying the
formation of subsidence craters were carried out, beginning in the
winter of 1969 (see table 3).
The first two explosions, in Holes 2-T
and 6-T, at scaled depths of burial of 130 and 113 m/kt1/3,
respectively, produced subsidence craters with radii somewhat larger
but not inconsistent with U.S. experience.
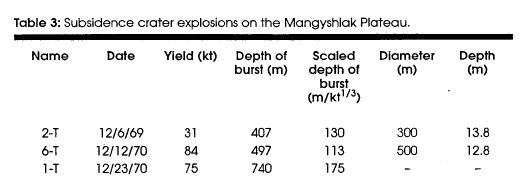
The crater depths were significantly
smaller than subsidence crater depths in U.S. experience.
These differences could well be the
result of differences in the physical properties of the media
between the explosion and the ground surface. The lack of a crater
for the explosion in Hole 1-T, whose scaled depth of burial was
almost 50 percent greater than 6-T, is not surprising and also
consistent with U.S. experience.
Even though these craters had volumes of more than 500,000 m3,
there is no reported attempt to use them for water storage or any
other use. There was also no further experimentation or application
of nuclear excavation to the creation of water storage reservoirs.
The explosions were completely contained without leakage, and
radiation levels in the area are reported to be at background. The
site is closed.33
In recent years, there have been several unconfirmed newspaper
reports that the actual purpose of these three explosions on the
Mangyshlak Plateau was a search for a new test site capable of
testing megaton-scale nuclear weapons.34,35 The history of Soviet
weapons testing would appear to be consistent with such a scenario.
In the late 1960s and early 1970s, both the U.S. and the Soviet
Union were developing megaton-scale warheads for the new generations
of heavy missiles, and a need existed for a high-yield test site.
At STS, the relative proximity of the
large city of Semipalatinsk limited the yields that could be fired
without significant seismic damage to buildings in that city. At the
Novaya Zemlya Test Site, at the north end of Southern Novaya Zemlya
along the Matochkin Shar Strait, nuclear devices were emplaced in
tunnels.
Terrain and permafrost significantly
limited the maximum yields that could be fired without venting to
the atmosphere. If the Mangyshlak Plateau was indeed a candidate,
the three tests carried out there would appear to be reasonable
candidates to explore its suitability for high yields.
Presumably, the site proved unsuitable, perhaps because of the
proximity (230 km) of the large city and breeder reactor facility at
Shevchenko on the shore of the Caspian Sea.
In 1972, a year and a half after the
last explosion on the Mangyshlak Plateau, the Soviet military opened
a new test site at the southern end the Novaya Zemlya at Chernaya
Bay with a small weapons test, followed a year later with several
high-yield tests, including a multi-megaton explosion on October 27,
1973. Over the next two years, they fired three more high-yield
nuclear weapons tests at this site.
The 150-kt limitation of the TTBT, which
became effective in April of 1976, precluded the necessity for a
high-yield site, and no further tests occurred at this new test
site.
Kama-Pechora Canal Project
Soon after the first two excavation explosions in 1965, another
project became the primary focus of the Soviet nuclear excavation
program - the construction of a canal to divert water from the
Arctic region into the Volga River basin and Caspian Sea.
Stimulated by a steady decline in the
level of the Caspian Sea over the preceding 35 years as a result of
climatic anomalies and municipal and agricultural uses of water from
the Volga-Kama River system, a number of water management agencies
in the Soviet Union had proposed diversion of water from the Pechora
River in the Komi Republic, which flows northward into the Barent
and Kara Seas, through a 112-km-long canal into the Kama and thence
south to the Volga River and the Caspian Sea.36, 37
Perhaps driven to compete with U.S. proposals to use nuclear
excavation to construct a new sea-level canal to replace the Panama
Canal, Soviet PNE program scientists proposed to use nuclear
explosions to construct the central 65 km of the Pechora-Kama canal
where it passes though higher elevations. Their proposals envisaged
the use of several hundred nuclear explosives, firing up to twenty
at one time with aggregate yields of as much as 3 Mt.
Preliminary cost estimates indicated
that the use of nuclear excavation would reduce the cost of the
canal by a factor of 2 to 3 compared to usual construction methods.
Tel'Kem-1 and Tel'Kem-2
As an initial step in
considering the use of nuclear excavation for this project, Soviet
scientists carried out a pair of cratering experiments in the
TelTtem area on the southeastern corner of the STS (see section A.2,
Appendix B).
The first, "TelTcem-l" on October 21,
1968, was a 0.24-kt explosion at optimum depth for cratering in a
saturated quartzose sandstone (35 m). The second, "Tel'kem-2" on
November 12, 1968, consisted of three 0.24-kt explosives in a row,
40 m apart, at the same depth-of-burst as TeTkem-1.
As expected, Tel'kem-2 produced a linear crater 142 m long, 60-70 m
wide, and 16 m deep, about 20 to 30 percent narrower and about 25
percent shallower than expected on the basis of Tellcem-l. However,
when judged relative to the "Sary-Uzen'" crater, Tel'kem-2 has about
the expected dimensions.
The results were also quite consistent
with the U.S. Buggy row-charge cratering explosion when
consideration is given to the differences in the geological media.
Radiation levels on the lip are reported to be 30
µR/hr, only
slightly above regional background levels.
Beyond the limits of throw-out from the
crater, the levels are at regional background.38
Taiga
In the fall of 1969, the Soviet Government Planning Agency, GOSPLAN,
approved going forward with the Pechora-Kama Canal39, and planning
within the Soviet PNE Program took on a greater urgency.
One problem had been identified that
concerned PNE scientists. The northern 30 km of the section of the
canal being considered for nuclear excavation was described as
sandstone, siltstone, and argillite rock, media somewhat similar to
the cratering media at STS. However, the southern 35 km portion was
largely saturated alluvial deposits, which could present difficult
slope stability problems.
Because of concerns over the stability
of the slopes of a nuclear canal in this portion of the canal, the
Ministry of Reclamation and Water Resources sponsored a nuclear
row-charge experiment code-named Taiga" for the southern end of the
portion being considered for nuclear excavation. The site was about
100 km north of the city of Krasnovishersk in the Perm Oblast'.
The alluvial deposits in this area
varied in depth between 90 and 130 m, being underlain by sandstone
and argillites and marl. The water table was reported to be from 5
to 17 m deep.
Three explosives with yields of 15 kt each were emplaced at depths
of about 127 m, roughly at the base of the alluvial deposits, to be
fired simultaneously. The scaled depths of burial were about 57 m/kt1/3-4,
which placed them somewhat deeper than optimum. The spacing between
the explosives was about 165 m, a spacing expected to enhance crater
width by about 10 percent compared to a single crater diameter.
The explosives used for the Taiga experiment were of a special
design in which the fission yield had been significantly reduced
over that used for the "Chagan" event in January 1965.
The design used was tested in Hole 125
at the Sary-Uzen' portion of the STS on November 4, 1970 40,41
several months before the Taiga event. Although specific details of
the explosives used for Taiga have not been provided, MinAtom has
reported that special nuclear explosives for excavation were
developed in the 1970s in which the fission contribution was reduced
to about 0.3 kt with the remainder of the energy coming from
thermonuclear reactions.42
The Taiga explosion was carried out on February 23, 1971, about 100
km north of the city of Krasnovishersk in the Perm Oblast'. It
produced a row crater about 700 m long and 340 m wide, almost 50
percent larger than expected. However, its depth was only about
10-15 m.
The final pan-shaped configuration was
the result of extensive failure of the saturated alluvial slopes.
Figure 3 is a sketch map of the crater and the surrounding lip and
throw-out area. The final crater slopes stabilized at an angle of
about 8-10 degrees.43
Although Soviet scientists remained
optimistic in interpreting the results of the experiment, by almost
any measure, the results of Taiga indicated that nuclear excavation
was probably not appropriate for the southern portion of the canal.
Even though the Soviets used a new, low-fission explosive for the
Taiga experiment that produced up to an order-of-magnitude less
fission product radioactivity than the one used for the Chagan
experiment, it was detected outside the Soviet Union by several
countries, including the U.S. and Sweden, who lodged protests
regarding violation of the LTBT. On site, the dose rate on the
crater lip at about one hour after the explosion was 50-200
µR/hr.
Eight days after the explosion, at
distances up to 8 km downwind from the crater, the dose rates were
23-25 µR/hr, only about twice natural background for the European
part of Russia. Within the fallout pattern, the contour representing
an accumulated dose of 0.5 Rem over the first year (about twice
background) extended some 25 km.44,45
Today, the general dose rate on the lip is 40-200
µR/hr with
isolated hot spots of up to 1mR/hr. On the surface of the water, the
dose rate is about 50 µR/hr. Radiation levels in the crater area
are determined by the long-lived radionuclides Co-60, Sr-137, Sr-90,
and tritium. In the lake, all the gamma-radiation emitters, Sr-90,
and tritium are below standards for drinking water. A restricted
area has been established within 2-300 meters of the crater, beyond
which the radiation levels are reported to be at background levels
and undetectable in vegetation 46,47
The disappointing technical results of the Taiga experiment, in and
of themselves, did not appear to discourage Soviet interest in using
nuclear excavation for this project. For some time, Soviet
scientists continued to discuss the project in general, and the use
of nuclear excavation in particular.
For example, the results of the Taiga
experiment were presented at the fourth IAEA meeting on PNEs in
January, 1975, together with a restatement of plans to proceed with
the project.48
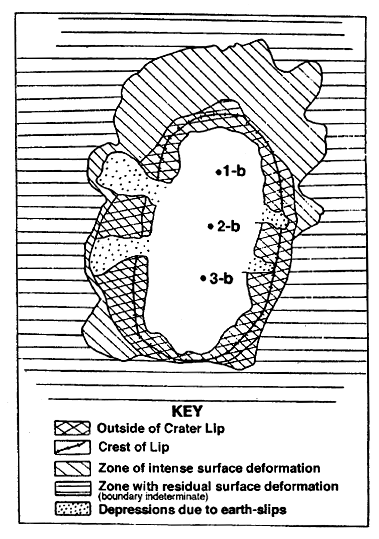
Figure 3:
Sketch map of the
"Taiga" Crater along the proposed alignment of the Kama-Pechora
Canal
showing the outline
of the crater and areas where there was failure of the crater slopes
(from endnote 36).
One of the principle motivations behind
the Soviet interest in having U.S./Soviet PNE bilateral discussions
in the early 1970s derived from their desire to jointly develop
general health and safety guidelines for carrying out PNEs such as
those for the Kama-Pechora Canal.
They also wished to use this interaction
to develop an "understanding" with the U.S. on how such large-scale
cratering projects could be rationalized to be in conformance with
the LTBT limitation on radioactivity from nuclear explosions across
national borders. Although, there were differences in the English
and Russian text of the LTBT that were less restrictive in Russian,
it was clear that a project of the magnitude of Kama-Pechora could
not be done without some "understanding" or perhaps modification of
the LTBT.49
Another indication of their continuing interest in the project was
the Soviet insistence that the Peaceful Nuclear Explosions Treaty,
which was negotiated in 1975-76 as a companion to the Threshold Test
Ban Treaty limiting nuclear weapons tests to 150 kt, permitted
aggregate yields for PNEs that were significantly greater than 150
kt.50
The Kama-Pechora Canal project was
repeatedly cited as the raison d'etre for this provision.
However, in the late 1970s and early 1980s, opposition began to
develop in academic and governmental circles to many of the
large-scale water diversion projects that were being proposed by the
Soviet government. Primary concerns were about the environmental
effects and possible climatological and hydro-logic changes
resulting from transfer of significant volumes of water from the
Arctic to the southern portion of the country.51
By the mid-1980s, these plans were
largely abandoned, including any use of nuclear excavation for the
Pechora-Kama Canal.
Dam
Construction
Crystal
Several' years after the Taiga explosion, on October 10,1974,
Soviets scientists carried out another small-excavation type nuclear
explosion 3 km northeast of the small settlement of Udachnyy in
Yakutia in Siberia and 90 km northeast of the town of Aikhal.
This explosion was under the sponsorship
of the Ministry of Light Metallurgy and a local combine called "Yakutalmaz,"
a diamond-mining enterprise. The purpose of the explosion was to
create a small dam in the Deldyn river. This area in Siberia is in
the permafrost region, and the rivers and streams only flow for a
few months in the summer.
The intent was to produce a small lake
that would retain the tailings from the diamond mine for subsequent
enrichment by Yakutalmaz (see section A.3, Appendix B).
For this experiment, a 1.7-kt explosive was placed at a depth of 98
m, a scaled depth-of-burst about twice that of Chagan and Sary-Uzen'.
The explosion produced a dome-shaped mound with a diameter of 180 m,
which rose to a height of 60 m and then settled back to an average
height of 10 m above the original surface.52
It is reported that the dome did not
rupture, and all the refractory radionuclides were contained
underground, although there would have been escape of gaseous
radionuclides such as Cs-137, Xe-133, Kr-85, and perhaps tritium
through fractures in the dome. There is no information regarding the
use of the "dam" or the lake behind it for its intended purpose.53
Gamma-radiation surveys in 1990 reported the general level was 1530
µR/hr with a peak level of 110
µR/hr after the dam was covered by
a meter of soil.54
Another source reports radiation levels
in 1991 on the uplifted dome to be predominantly background levels
(9-15
µR/hr) except for a limited area northeast of the dome where
it reached a level of 50-60
µR/hr. After it was covered with 6 m of
rock from a nearby quarry, the level dropped to background level.
No radionuclides were detected in water
samples taken from the dam, drilling or earthwork in the dam, or
within 100 m of the dam.55
Lazurite
A few months later on December 7,1974, Soviet scientists carried out
a second experiment with a deeply buried cratering explosion on the
edge of the STS, a few kilometers south of the Sary Uzen' crater.
Their intent was to produce an upthrust
dome similar to Crystal on a slope that would subsequently slide
down the slope and form a landslide dam, similar to what Soviet
engineers had done with large chemical explosions on a number of
occasions, most notably near Alma Ata in Kazakhstan and on the
Vakhsh River in Tadzhikistan. 56
For the "Lazurite" experiment, a 1.7-kt explosive was placed under a
20° slope consisting of quartzite and flinty slate. It was
positioned 75 m from the slope in a vertical direction and 70 m in
the line of least distance to the slope. The scaled depth of burst
was about 5-10 percent greater than that for the U.S. explosion
Sulky, which produced a mound of broken rock.
The Lazurite explosion also produced a mound of broken rock, which
had a diameter of 200 m and a height of 14 m. No description of the
dam that was formed by Lazurite has been published. There was very
little venting, and radiation levels on the mound immediately after
the explosion were reported to be three to four orders of magnitude
less than for cratering explosions such as Sary Uzen'.57
Radiation levels today are described as
being at background, but monitoring of the site is continuing.58
The Soviet interest in nuclear excavation appeared to come to an end
with the Lazurite explosion. All tests after Lazurite were designed
to be completely contained explosions.
However, as mentioned above, their
planning and public positions continued through the mid-1970s to
include strong emphasis on nuclear excavation and the Kama-Pechora
Canal.
Contained
Applications
Stimulation of Oil and Gas Production
While MinAtom's Program No. 7 for the "Utilization of Nuclear
Explosions in the National Economy" was planning the nuclear
excavation program and the Chagan cratering explosion, the Soviets
also began to consider the use of PNEs for various industrial
applications.
The first area to receive serious study
was the application of nuclear explosions for the stimulation of oil
production, carried out in cooperation with the Ministry of Oil
Production (see section B.l, Appendix B).
Experience has shown that the efficiency of recovering oil from
carbonate oil reservoirs is, in general, fairly low (<40 percent).
Several secondary recovery techniques are practiced with varying
degrees of success, depending on the nature of the reservoir and the
character of the oil.
These methods include water and gas
injection, fire or hot-water flooding, hydrofracturing to increase
the permeability of the formation, and the introduction of gas into
the oil to reduce its viscosity.
However, many fields resist such
conventional techniques.
Butane
The first oil field considered for treatment was the Grachevka
reservoir, located about 150 km north of the city of Orenburg in the
Bashkir Republic, near the southern end of the Ural Mountains.
This formation has been described as a
solution gas drive reservoir in which ultimate recovery had been
projected as ~25 percent of the in-place resources. The reservoir is
a limestone reef at a depth of 1,000-1,500 m overlain by interbedded
anhydrite and halite layers, which formed a "gas cap." The
oil-producing section is isolated from an underlying pressurized
water zone by a bitumenized or oxidized layer 25-50 m thick.59
Production from this reservoir in the first few years after it was
opened increased rapidly to several hundred tons of oil per month as
new holes were drilled into the reservoir. But after three years,
production began to sharply decrease as the gas/oil ratio began to
rise significantly.
By the seventh year, production was down
to about 100 tons/month, and the gas/oil ratio had risen from 100 to
500 m3 of gas/ton of oil. The apparent cause was the development of
channeling and the escape of dissolved gas from the deposit, leaving
the oil behind. To reverse these trends, Program No. 7 and the Oil
Production Ministry decided to make their first attempt at nuclear
stimulation with this field.
The first stage of the "Butane" project involved the simultaneous
detonation on March 30, 1965, of two 2.3-kt nuclear explosions about
200 m apart at depths of 1,375 and 1,341 m in the Grachevka
formation.
This depth placed the explosions and the
associated collapse chimney totally within the oil deposit. A second
explosion, which was a single 7.6-kt nuclear explosion at a depth of
1,350 m and located 350 m west of one of the two 2.3-kt explosions,
was carried out later that year on June 6, 1965.60 Because of the
small yield and perhaps the porous carbonate rock, these explosions
were not detected by world-wide seismic networks.
The locations given in Appendix A are
based on the geographical location provided in endnote 15. The
devices used for this experiment have been described as specially
designed and tested for this application.61
Over the next few years, increased production was reported for some
20 wells within 300-470 m of the stimulation wells. The oil/gas
ratio dropped sharply, and oil production increased about 40 percent
over previous projections. Results available to date indicate that
the stimulation will increase the ultimate recovery from the
formation by 40-50 percent. Table 4 summarizes the results of the
first 8 years of production from the Butane project as well as the
results of other oil and gas stimulation projects in comparison with
the U.S. gas stimulation projects.62
The Butane explosions were apparently completely contained, and
radiation levels in the area are reported to be at background
levels. Within the collapse chimney region, tritium produced by the
nuclear explosions diffused into the gas cap above the oil-bearing
horizons and appeared in gas produced with the oil. Initially the
average tritium level in the gas from the field was measured at 0.03
uCi/liter.
Within 3 years, it had stabilized at
about 0.003 uCi/liter. Tritium levels in the oil were less than 3
uCi/liter, which is approximately the same level as in the gas on a
unit weight basis. Only trace amounts (<0.1 uCi/liter) of the
fission product radionuclides Cs-137 and Sr-90 were reported.63,64
On June 16 and 25 in 1980, two additional 3.0-kt explosions
were carried out in the Butane reservoir at depths of about 1,400 m.
These two explosions were also not detected by the world-wide
seismic networks, and the locations given in Appendix A are based on
geographic information in endnote 15.
No additional information has been made
available on the specific purpose or results of these explosions.
Grifon
Four years after the first Butane explosions, the Soviet Oil
Ministry sponsored stimulation of another oil reservoir, but of a
different type.
The second effort, project "Grifon,"
sought to stimulate the Osinskii deposit, 100 km southwest of the
city of Perm on the western slope of the Urals. The Osinskii
reservoir was a carbonate reef deposit being produced through the
use of water injection into wells surrounding the oil reservoir.65,66
On September 8 and 26 in 1969, two 7.6-kt explosions were fired
1,200 m apart at depths of 1,212 and 1,208 m in the middle of this
reservoir.
At these depths, the explosions were about 70 m below the
water-oil contact at the base of the reservoir. The collapse
chimneys would have been expected to extend 50-80 m into the
oil-bearing formation. Although little production data have been
made available, Soviet scientists stated that early results
indicated an increase of 30-60 percent in production.67
As shown in table 4, results indicate a
production increase of 50-60 percent over that for the previous 20
years.
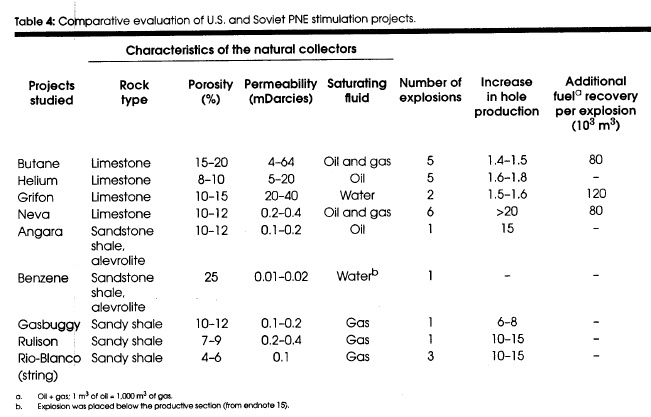
The Grifon explosions were completely contained, but post-shot
drilling into the chimney region and removal of water from the
explosion region led to contamination of the surface area and
equipment that was later decontaminated, and the site is reported to
be at background levels.
The primary radiation hazard to production
from this site is associated with the presence of Cs-137, Sr-90, and
tritium in the water underlying the oil that is being used to
pressurize the field. However, radioactivity levels in oil produced
from the field are reported by MinAtom to be similar to those
reported for the Butane field.
However, there are reports in the Russian press that describe a less
optimistic situation. A recent article by Academician Yanshin states
that studies of the environmental effects of PNEs in the Perm area
in 1991-92 indicated that by 1978, radionuclides Cs-137 and Sr-90
began to appear in holes near the explosion sites.68
Over the next ten years, the area of
contamination had spread to some 65 production holes and represented
a threat of contaminating the nearby Votkinsk water reservoir, the
Kama River, and even the Volga River basin. In the region of the
emplacement holes, radiation levels were reported to be about 60
µR/hr
and at specific injection holes 20-50
µR/hr.
In some areas, radiation levels were
reported to be as high as 3 mR/hr.69'70 There are reports that the
refineries in Perm have refused to accept oil from the Osin field,
and several holes have been closed.71
MinAtom confirms that oil from the
Grifon site is not acceptable to regional refineries.72
Project Takhta-Kagulta
One month after the first two Grifon oil stimulation explosions, on
September 26,1969, the Soviet PNE program, with the sponsorship of
the Soviet Ministry of Gas Production, carried out their first
effort directed at stimulating the production of gas from
low-permeability gas reservoirs.
The site of this test was in the
Takhta-Kagulta gas field in southern Russia on the northern slopes
of the Caucasus Mountains about 90 km northeast of the city of
Stavropol'.
The Takhta-Kagulta gas field is a very large geologic structure in
which the production horizon is a very thin 5 to 13-m-thick section
consisting of clayey siltstone lying at a depth of about 700-750
m.73
The test was a single 10-kt nuclear explosion at a depth of 712
m.
During the second U.S./U.S.S.R. Bilateral Meeting in February 1970,
Soviet scientists revealed to the Americans that such an experiment
had been carried out, but no additional information on the results
were made available - at that time or since.
Moreover, this was the only test
reported to have been sponsored by the Gas Ministry. The extremely
thin nature of the production horizon would appear to make this a
poor candidate for nuclear stimulation since the collapse chimney
would be expected to be 5-10 times taller than the thickness of the
deposit. The lack of information on the results of this test would
appear to confirm a very disappointing result.
No escape of radioactivity has been
reported, and the site is closed.74
Project Neva
In the fall of 1976, the Soviet PNE program, this time with the
sponsorship of the Ministry of Geology, began a new gas stimulation
project, subsequently referred to as the Neva project.
This project consisted of a series of
explosions in a hydrocarbon reservoir at a site located about 120 km
south-southwest of the Siberian town of Mirnyy in the Siberian Yakut
Republic. The producing formation at the Neva site, named the
Sredne-Botuobinsk reservoir, consists of a dolomite and limestone
section capped by a salt layer at a depth of 1,500-1,600 m
containing both oil and gas, although prior to nuclear stimulation,
it was only considered for gas production.75
At the time of the first experiment, code-named "Oka," on November
5, 1976, the field was under active development. The Oka explosion
was 15 kt at a depth of 1,522 m. Initially, production tests several
months after the explosion were conducted in a pre-existing
exploratory hole 120 m from the Oka hole.
Whereas preshot gas production from this
hole had been 3,0005,000 m3/day, periodic post-shot
production over a 75-day period produced over 100,000 m3/day as well
as 20-22 m3/day of oil. Production testing from the Oka emplacement
hole over a three and a half month period resulted in over 100,000
m3/day of gas, but no oil which was accumulated in the central
chimney region.
At the end of the test period, the flow
rate was still 50,000 m3/day.
The second explosion in the Sredne-Botuobinsk formation, code-named
"Vyatka," was carried out on October 7, 1978, near Oka. It had a
yield and depth of burial similar to Oka and was also 120 m from
another exploratory hole, which had no significant production before
Vyatka. Post-shot production from the Vyatka emplacement hole
averaged 60,000 m3/day over a two-month period and was
still 38,000 m3/day at the end of the testing period.
76
The third explosion, "Sheksna," was fired exactly one year later on
October 7, 1979, with about the same yield but about 50-70 m deeper
than Oka and Vyatka. No specific results of production testing in
the vicinity of Sheksna have been provided.
Three more explosions, Neva-1, Neva-2, and Neva-3, were carried in
1982 and 1987 to extend the stimulation of the Sredne-Botuobinsk
formation over a - larger area. In November, 1987, the Soviets
carried out the seventh and last explosion at the Sredne-Botuobinsk
site, "Neva-4," which MinAtom lists as a stimulation explosion.
However, the yield of the explosion, 3.2
kt, and its depth of 815 m in a salt formation would suggest that
this explosion was, in reality, a storage cavity used to dispose of
radioactive and toxic wastes generated by the stimulation and
production activities at the site. This is supported by the fact
that table 4 from endnote 15 lists only 6 stimulation explosions at
the Neva site.
The overall results of stimulation at the Neva site are shown in
table 4 in comparison with other U.S.S.R. and U.S. stimulation
projects. It shows that the permeability of the Sredne-Botuobinsk
reservoir was comparable with that of the U.S. Gasbuggy and Rulison
reservoirs, but the results of stimulation were about 2-3 times
greater than those realized in the U.S. experiments.
This difference could well be due the
fact that the Sredne-Botuobinsk reservoir was limestone and
dolomite, whereas the American reservoirs were primarily shale.
Based on the fact that only four out of forty exploratory holes had
commercial production potential, nuclear stimulation has made the
field commercially valuable. The value of the nuclear stimulation of
the field was calculated to be worth 100,000,000 rubles (1980).
In addition, whereas the field was
regarded as only a gas field prior to stimulation, commercially
significant volumes of oil were recovered from the field.77
Along with the mechanical alteration of the rock surrounding the
explosion, Soviet scientists also report the discovery of a new
phenomena, the permanent electrical polarization of the rock (see
figure 4). The region of anomalous polarization extends to distances
of 200-250 m/kt1/3 from the explosion. It is directed
toward the explosion point and facilitates the motion of oil toward
the center of the explosion.
This phenomenon was first noted during
work on the Neva project. Studies indicated that the strength of the
polarization is dependent on the properties of the rock and is
significant only for low-permeability reservoirs. There are no
reports on whether this phenomenon was looked for or found at the
other stimulation sites.78,79
MinAtom reports that all seven explosions carried out at this
site were totally contained. Access to the emplacement holes has
been closed, and they have been cemented (perhaps with the exception
of Neva-4). Work at the site could be resumed.
Radiation levels at the site are at
background levels.80
Project Helium
In 1981, the Soviet PNE
program began a new oil stimulation project called Project "Helium"
in the Tyazhskii carbonate oil reservoir near the town of
Krasnovishersk on the western slope of the Urals, about 800 km north
of the first oil stimulation project, Butane.
The characteristics of the formation and
the technology used for producing the field are described as being
very similar to those at Butane.
The first explosion on September 2, 1981, was a 3.2-kt explosion
placed at a depth of 2,088 m, below the oil-bearing section. The
second and third explosions were carried out three years later on
August 28, 1984, timed to be five minutes apart.
The yields and
depths were similar to those for the first explosion.
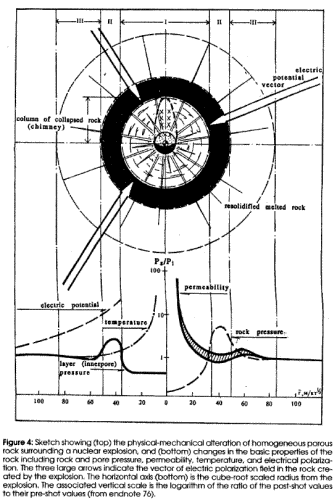
The last two
explosions in Project "Helium" were fired about three years later on
April 19, 1987, again five minutes apart with similar yields and
depths of burial.
Available results of production testing
are limited to the numbers in table 4, which indicate a production
increase of 60-80 percent after the stimulation explosions.
All of the explosions were reported to
have been successfully contained, and the site is under active
exploitation.81
Projects Angara and Benzene
The Soviets acknowledge carrying out two additional oil stimulation
explosions, Projects "Angara" and "Benzene," both of which were in
western Siberia in the middle of the great Ob' River basin.
The first, Project "Angara," was
sponsored by the Geology Ministry and was carried out on December
10,1980, in the Yesi-Yegovskii oil field. It employed a 15-kt
explosion at a depth of 2,485 m. The second, Project Benzene, was
sponsored by the Oil Ministry and was fired on June 18, 1985, in the
Sredne-Balykskii oil field. It was a 2.5-kt explosion at a depth of
2,859 m.
No data have been published on the
results of these projects, except that the Angara site is closed,
but the Benzene site is under active development.
Both were successfully contained.82
Summary of Oil and Gas Stimulation
Overall, the Soviet PNE program carried out 5 projects directed at
the stimulation of oil production, all from limestone or dolomite
reservoirs.
Three of the projects utilized multiple
explosions with yields between 2.3 and 7.6 kt. Results over 10-20
year production periods indicate an increase in production of about
40-80 percent over that projected for the fields before stimulation.
Two oil stimulation projects using a single explosion have been
carried out, but no results have been published.
All of the explosions were completely
contained, and no problems with radioactive contamination of the
site or product have been reported except for Project Grifon, where
product and ground water contamination appears to be a growing
problem.
The Soviet program to stimulate the production of natural gas
appears to have consisted of two projects, only one of which
produced results that have been reported. This project, "Neva,"
utilized six 13 to 16-kt explosions for stimulating a dolomite
reservoir and one 3.2-kt explosion in salt, presumably for storage
or disposal of waste.
Results over a 15-year production history
indicate a recovery rate more than 20 times normal, results about 2
times more favorable than those for U.S. experience. All explosions
were contained. Although the gas would be expected to contain a low
level of tritium, no problems with product contamination have been
reported.
As was done by the U.S. Plowshare Program, special explosives were
developed by the Soviet weapons laboratories to meet the unique
requirements of stimulating oil and gas reservoirs.
The great depths of such reservoirs
(1,000-2,000 m) place great value on reducing the diameters of the
explosives and building them so they can withstand the elevated
pressures and temperatures experienced at those depths. There is
also a great premium on reducing the tritium produced in the
explosive and the surrounding medium to reduce problems of
contaminating hydrocarbon products with tritium.
To meet these requirements, the Soviets
developed special explosives with a 30 to 60 cm diameter and the
appropriate yields capable of withstanding the emplacement and rough
transport conditions.83
Mention should be made of a magnitude 4.4 seismic event at 9:00:06
GMT on July 19, 1982, 120 km northeast of Kotlas in the southeast
corner of the Komi Republic. Russian geoscientists in the Schmidt
Institute and the Institute for Dynamics of the Geosphere have
associated this event with the Soviet PNE program and have referred
to it by the name "Komipetroleum" indicating their belief that its
purpose was oil stimulation 84
Publications by the MinAtom make no
reference to this event.
Cavity
Technology Development
Project Halite
Early in the development of the Soviet
PNE program, perhaps in reaction to the earlier U.S. explosions in
salt that produced standing cavities Gnome and Salmon, the decision
was made to develop the technology of creating cavities in salt.
They chose a site about 180 km north of
the city of Astrakhan at the north end of the Caspian Sea. The site
for these studies was near the small village of Azgir in the middle
of a large semi-desert area. The geologic medium at Azgir consisted
of two large salt diapirs, the Western and Eastern Azgir Domes, that
go to great depth but with relatively thin alluvial cover (5200 m).
The water table in the area is fairly
shallow, near the top of the salt formation.85
A-l
The first experiment in this program, code-named "Halite," was A-l,
a 1.1-kt explosion fired on April 22, 1966, in the Western Azgir
Dome at a depth of 161 m, a scaled depth of about 155 m/kt1/3.
It produced a cavity about 25 m in diameter and a volume of 11,200 m3.
As with all nuclear explosions in salt that produce cavities,
radioactive debris from the explosion initially is embedded in the
walls of the cavity that are covered with molten salt. Within a few
minutes after the explosion, the molten salt on the walls runs to
the bottom of the cavity, carrying the refractory radioactive debris
with the salt.
The molten salt forms a segment-shaped
puddle at the bottom of the cavity about a third of a cavity radius
deep at the center, which ultimately solidifies, trapping the
refractory radioactive debris in the resolidified salt. Gaseous
radionuclides appear in the gases filling the cavity.
Shortly after the explosion, the A-l cavity began filling with
water, apparently the result of poor sealing of the emplacement hole
and extensive fracturing above the cavity by spalling of the ground
surface. The roof also collapsed some 8,000 m3 of rock salt into the
cavity, hampering efforts to study the cavity.
In addition, gaseous radioactivity from
the cavity escaped into nearby instrument holes and leaked to the
surface, contaminating the local environment.
A-2
The second Halite explosion, A-2, was carried out two years later on
July 7, 1968, in the same Western Azgir Salt Dome but about 8 km
north of A-l at a somewhat greater scaled depth.
It consisted of a 27-kt explosion at a
depth of 597 m, a scaled depth of burial of about 200 m/kt1/3.
It produced a spherical cavity with a radius of about 32 m and a
volume of about 140,000 m3. This cavity also began to leak around
the emplacement hole and ultimately filled with water, but there was
no early vent of gaseous radionuclides.
A-l and A-2 were thoroughly explored and provided very useful
experience in making and working with explosion cavities in salt
that led to ideas for using such cavities for a number of
applications. A-2 was repeatedly used in a series of experiments
designed to produce transplutonic elements (as discussed below).
A-3
The third explosion in the Halite series, A-3, was carried out three
years later on December 22,1971, in the Eastern Azgir Salt Dome
about 16 km east of A-l and A-2.
This time, the yield was 64 kt, and
the depth-of-burial 986 m for a scaled depth-of-burial of about 245
m/kt11/3. The explosion produced a spheroidal cavity that had a
horizontal radius of 38 m and a vertical radius of about 33 m. The
radius of an equivalent sphere would be 36.2 m.
The cavity remained
dry and was subsequently used for a seismic decoupling experiment
(see below).86
Extinguishing Runaway Gas Well Fires
Shortly after the Soviet PNE Program was established, an urgent
industrial problem was brought to the leaders of the program - could
an underground nuclear explosion be used to put out a gas well fire
that had been raging for some 3 years? (See section B.2, Appendix
B.)
Urtabulak
On December 1,1963, while drilling gas Well No. 11 in the Urtabulak
gas field in Southern Uzbekistan about 80 km southeast of Bukhara,
control of the well was lost at a depth of 2450 m. This resulted in
the loss of more than 12 million m3 of gas per day through an 8-inch
casing, enough gas to supply the needs of a large city, such as St.
Petersburg. Formation pressures were about 270-300 atmospheres.87-88-89
Over the next three years, many attempts were made using a variety
of techniques to cap the well at the surface or to reduce the flow
and extinguish the flames. However, because the bottom 1,000 m of
the casing had not yet been cemented, such attempts led to diversion
of the gas into nearby wells and to serious personnel safety
problems because of the high H2S content of the gas.
Underground
attempts were hampered by the fact that the location of the lower
portion of the hole had not been logged at the time control was
lost.
Finally, in the fall of 1966, a decision was made to attempt closing
the well with the use of a nuclear explosive. It was believed that a
nuclear explosion would squeeze close any hole located within 25-50
m of the explosion, depending on the yield. Two 44.5-cm (13.5 in)
diameter slant wells, Holes No. 1c and 2c, were drilled
simultaneously.
They were aimed to come as close as possible to Hole
No. 11 at a depth of about 1,500 m in the middle of a 200-m-thick
clay zone. This depth was considered sufficient to contain the
300-atmosphere pressure in the gas formation below. A number of
acoustic and electromagnetic techniques were used to estimate the
distance between Hole No 11 and inclined explosive emplacement hole
at 1,450 m.
The final estimate for the closest distance between Hole
No. 11 and Hole No. 1c was 35
±
10 m.
The location for the explosive in Hole 1c was cooled to bring it
down to a temperature the explosive could withstand. A special 30-kt
nuclear explosive developed by the Arzamas nuclear weapons
laboratory for this event was emplaced in Hole 1c and stemmed. It
was detonated on September 30, 1966.
Twenty-three seconds later the
flame went out, and the well was sealed.90
Pamuk
A few months after the closure of the Urtabulak No. 11 hole, control
was lost of another high-pressure well in a similar nearby field,
Hole No. 2-R in the Pamuk gas field.
In this case, drilling had
progressed to a depth of 2,748 m before the gas-containing horizon
was encountered, and gas pressures were significantly higher than
those at Urtabulak (580 atm). A month and a half after the runaway
well started, it blocked itself at a depth of 800-1,000. Remedial
work was done in the well and appeared to have resolved the problem
when, four months later, gas started coming to the surface through
other holes and through the ground itself.
After several unsuccessful attempts to seal the well by hydraulic
fracturing from a slant-drilled well, it was decided to again use a
nuclear explosive to pinch off the runaway well. A new inclined
hole, No. 10-N, was drilled to intersect Hole 2-R in the middle of a
salt formation that overlay the gas producing formation.
Measurements after it had been drilled indicated that the minimum
separation distance at a depth of 2,440 m was 30
± 5 m.
This time, a special explosive developed by the Chelyabinsk nuclear
weapons laboratory was used, one that had been designed and tested
to withstand the high pressures and temperatures in excess of 100°C
expected in the emplacement hole. It also was designed to be only 24
cm in diameter and about 3 m long to facilitate its use in
conventional gas and oil field holes. Its yield was 47 kt.91
The explosive was inserted into Hole 10-N and detonated on May 21,
1968, at a depth of 2,440 m. Because of the large amount of gas that
had infiltrated the overlying strata during the preceding two years,
the flow continued for seven days before it finally died out and the
seal was complete.
The second "success" gave Soviet scientists great
confidence in the use of this new technique for rapidly and
effectively controlling runaway gas and oil wells.
Crater and Fakel
Some four years later, two
more opportunities arose for the use of nuclear explosions to
extinguish runaway gas well fires.
The first, code-named "Crater,"
was in the Mayskii gas field about 30 km southeast of the city of
Mary in Central Asia. Control of the gas well was lost on May 11,
1970, and about 700,000 m3 of gas was lost per day. The producing
horizon in this field was at the 3,000-m level. No details have been
made public about this application, except that on April 11, 1972, a
14-kt explosion at a depth of 1,720 m in an argillite formation was
used to successfully seal the runaway well.
On July 7,1972, another runaway gas well in the Ukraine, about 20
north of the city of Krasnograd and 65 km southwest of Karkov, was
sealed with a nuclear explosion. The runaway well was in the
Krestishche gas formation at a depth of over 3,000 m. No
additional information has been made available except that for this
event, named "Fakel," a 3.8-kt explosion at a depth of
2,483 m in a salt formation, was used.
The small yield would
indicate that the location of the runaway well was well known, and
the explosive emplacement hole was drilled to be very close to it at
shot depth.
Pyrite
The last attempt to use this application occurred in 1981 on a
runaway well in the Kumzhinskiy gas deposit in the northern coast of
European Russia near the mouth of the Pechora River, 50 km north of
the city of Nar'yan Mar.
Control of the well was lost on November
28, 1980, resulting in a loss of about 2,600,000 m3 of gas per day.
On May 5, 1981, a 37.6-kt nuclear explosion, code-named "Pyrite,"
was detonated at a depth of 1,511 m in a sandstone-clay formation
near the runaway well. However, the nuclear explosion did not seal
the well, perhaps because of poor data on the position of the
runaway well.
No additional details have been published on the
results of the nuclear attempt or of subsequent efforts to close the
well by other means.92
Of the Soviet attempts to extinguish runaway gas wells, MinAtom
reports that all were completely contained, and no radioactivity
above background levels was detected at the surface of the ground
during post-shot surveys.93
Underground
Cavities for Storage of Gas Condensate
Building on their experience with creating the two cavities in salt
at Azgir in 1966 and 1968, Soviet scientists began to consider
possible use of such cavities within the industrial sectors for
underground storage.
In the late 1960s, contacts were established
with specialists at the Ministries of Oil, Gas, Chemistry and Oil
Refining to assess their future requirements for underground storage
and their interest in exploring the use of nuclear explosions to
help meet those needs.
The greatest interest was found in the Oil
Production Ministry, and plans were quickly developed for a program
to develop this application (see section B.4, Appendix B).
The experience at Azgir with the Halite A-l and A-2 explosions
clearly identified two of the most significant technical issues that
had to be dealt with: isolation of the cavity from access to any
source of water through fractures, cracks, or the emplacement or
other holes near the cavity; and finding a depth that would be great
enough to contain the required explosive yield without exacerbating
problems of cavity stability against collapse or compression by the
lithostatic pressure.
Any leakage of water into the cavity, as
occurred in both the early cavities at Azgir, could quickly lead to
leaching of the radioactivity trapped in the recongealed salt lens
at the bottom the cavity and contamination of any product stored in
the cavity.
Project Magistral
The first experiment
specifically directed at the use of underground nuclear explosion
cavities for storage, Project "Magistral'," was carried out in the
Sovkhoz gas deposit about 70 km northeast of Orenburg, and 100 km
south of the first oil stimulation project at the Butane site (see
figure 5).94
The Magistral explosion had a yield of 2.3 kt and was emplaced at a
depth of 702 m in a bedded salt formation. It was fired on June 25,
1970, and produced a cavity with a volume of 11,000 m3 (radius ≈ 14
m). After several months, the cavity was entered through the
emplacement hole, and after some 6 months it was filled with natural
gas from the nearby Sovkhoz gas field to a pressure of 8.4 MPa (84
atm).95-96
The Magistral cavity stayed in industrial use for the next 18 years.97
Deactivation and decontamination of the
site began in 1993 to clean up radiation levels reported to be 30-40
µR/hr in the
immediate vicinity.98 About 3,000 tons of soil have been
contaminated, to an average level of about 5 x 105 pCi, by spillage
while pumping radioactive solutions from the cavity.
Beyond the
industrial site, the radiation levels are described as "background."
Project Sapphire
Following the success of the
Magistral project, scientists from the Soviet PNE program and the
Gas Production Ministry turned their attention to another site about
100 km southwest of Magistral and 40 km south-southwest of Orenburg
(see figure 5).
The new site, Project "Sapphire," was a bedded salt
formation that overlay the Orenburg gas condensate deposit, one of
the largest in the Soviet Union at the time of its discovery in
1967.
Gas condensate is a very high-quality hydrocarbon that is a mixture
of hydrocarbon gases (propane, butane, and pentane) that are liquid
at high pressure. Gas condensate is quite valuable, but in some
fields it is contaminated with hydrogen-sulfide and dissolved gases,
which makes it very expensive to store on the surface, requiring
high-pressure corrosion-resistant metal tanks.
At Orenburg, the
hydrogen sulfide content was reported to be 2.7 percent.
It appeared
that storage in salt cavities would eliminate the corrosion problems
and permit the dissolved gas to outgas before the gas condensate was
sent to the refinery for processing. Moreover, additional storage
capacity was required because the initial capacity of the
gas-condensate processing plant at Orenburg did not match the peak
processing capability of the natural-gas processing plant.99
The first of two Sapphire explosions was detonated on October
22,1971, at a depth of 1,142 m in the middle of the bedded salt
formation. The gas producing formation was at a depth of 1,400-1,800
m.
The nuclear explosion with a yield of 15 kt produced a 50,000 m3
volume cavity.
Reentry was made through the emplacement hole, and
after flushing and cooling of the cavity with natural gas, the
cavity was tested to a pressure of 8.4 MPa (84 atm) for 30 days and
found to be sealed.
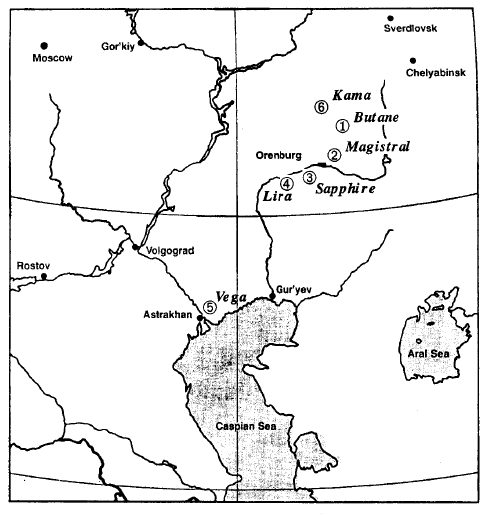
Figure 5
Map showing various PNE sites north of teh Caspian Sea.
1 - Butane is the firts oil stimulation project
2 - Magistral
3 - Saphire
4 - Lira and 5 - Vega are gas condensate storage projects
6 - Kama is the toxic waste storage project
A second explosion was detonated at the Sapphire site two years
later on September 9, 1972, a 10-kt explosion at a depth of 1,145 m,
again in the bedded salt formation. It produced a similar but
somewhat smaller cavity.
Both of these explosions preceded the construction of the
gas-processing complex, one of the largest in the world, that was
subsequently built at Orenburg by a consortium of western
engineering firms. It actually consists of three plants, each with a
capacity of processing 17 billion m3 per year.
Upon completion of
the first phase of the gas-processing complex in 1974, gas
condensate was introduced into the cavities, and the storage
reservoirs were turned over to industrial use. It was operated at a
working pressure of 8 MPa (80 atm) with the gas condensate being
removed by gas displacement.
Use of both of these sites was temporarily stopped in 1993 to permit
repair and decontamination work to clean up specific locations of
above-background radiation in the industrial area.
No contamination
of the products stored in the cavities has been reported. Beyond the
limits of the industrial area, radiation levels are reported to be
at background.100
Project Vega
In 1980, the Soviet PNE
Program and Gas Production Ministry began an extensive application
of the underground storage technology proven in Sapphire at a new
site about 700 km south of Sapphire, 40 km north-northeast of the
city of Astrakhan at the north end of the Caspian Sea (see figure
5).
This new project, called "Vega," was
located on the southern edge of the newly discovered Astrakhan
gas-condensate field, one of the largest gas-condensate fields
discovered in the Soviet Union, many times larger than the Orenburg
deposit.
The Astrakhan field had a very high level of
hydrogen-sulfide (25 percent), almost an order of magnitude greater
than that at Orenburg, contaminating the gas. In addition, the
Astrakhan field was reported to have very high levels of carbon
dioxide (12 percent) that had to be separated out before the natural
gas could be put in the distribution system.
The Astrakhan
gas-condensate reservoir occurs in a limestone formation at a depth
of about 4000 m and is overlain by the Sentovskii salt massif that
extends to about 500 m from the surface.101
The first explosion at Vega was carried out in the salt formation on
October 8, 1980, at a depth of 1,050 m. Its yield was 8.5 kt. The
following year on September 26, two explosions of similar yield were
carried out at about the same depth and with an interval of 4
minutes between explosions. A little over a year later, on October
16, 1982, four explosions were fired at Vega with an interval of 5
minutes between explosions. Russian sources give the yield for one
of the explosions as 13.5 kt with the rest being 8.5 kt.102
On
September 24,1983, six more explosions were carried out at the Vega
site at intervals of 5 minutes. The yields were all 8.5 kt at depths
of about 1,000 m.
On October 27, 1984, the last two explosions were fired at the
"Vega" site with a 5 minute interval between them, bringing the
total at the site to 15 explosions. The depths of burial for these
explosions were also 1,000 m, but their yields are given as 3.2 kt,
only about a third that of the earlier explosions. Considering the
very high sulfur content of the gas condensate and the size of the
deposit at Astrakhan, it is suggested that these later two cavities
may well be for waste storage or disposal.
The smaller yield of all the explosions at this site, in comparison
with the yields at the Orenburg deposit, are attributable to the
relative proximity of the large city of Astrakhan (>500,000
inhabitants) and numerous smaller towns and industrial facilities
built on the Volga River delta above the Caspian Sea, all of which
are susceptible to seismic damage.
Seismic limitations determined
the maximum single yield that was permitted, and the total storage
requirements of the Astrakhan field and processing plant dictated
the number of explosions. The use of several small explosions,
spaced a few minutes apart, rather that one larger explosion, would
allow the same storage volume to be produced with significantly
lower probability of seismic damage, minimal operational costs for
disruption and delay, and fewer evacuations of the local
populations.
The single explosion at 13.5 kt may have been a test to
see what seismic damage a larger yield would incur.
Assuming that the latter two explosions were for storage, the other
thirteen explosions would have produced a storage volume of about
400,000 m3, about four times the storage volume at Orenburg.
Beginning in 1986, seven of the cavities were filled with gas
condensate as part of industrial operations, two were used for waste
disposal, and six were placed in reserve.
The availability of these
cavities reportedly permitted start-up of the production and
processing of gas condensate from the Astrakhan field significantly
earlier than conventional storage would have permitted.
However, beginning in 1987, it was observed that the six empty
cavities had started to converge, losing up to 40 percent of their
volume. This was attributed to the absence of any counter-pressure in
the cavity and an anomalously high horizontal tectonic stress in
the salt massif.
Ultimately, the shrinkage led to destruction of the
spherical layer of salt on the surface of the cavities, collapse of
the walls into the cavity, and to fractures that allowed five of the
six cavities to fill with water. The decision was made to retire the
six cavities from use, and the access and other holes in the area
were sealed. It is reported that none of the radioactive brine in
the cavities has reached the surface.103
However, there is a
published report that water from the cavities has began to appear at
the surface as artesian springs containing radioactivity.104 All of
the fifteen Vega explosions were completely contained.
The areas
around some of the access holes are reported to have relatively
minor above-background contamination (30-40
µR/hr), but beyond the
industrial areas, radiation levels are at background.105 No
information has been provided on possible product contamination
levels.
Project Lira
Three years after beginning Project Vega at the Astrakhan
gas-condensate deposit, the Soviets initiated another industrial
application of the underground storage technology, Project "Lira,"
at the Karachaganak gas-condensate field located about 140 km east
of Uralsk and 130 km west of the city of Orenburg (see figure 5).
The field, which was discovered in 1979 and actively developed in
the early 1980s, is regarded as an extension of the Orenburg field
and is about the same size as the Orenburg field. As at Orenburg,
the natural gas and gas condensate contain high levels of hydrogen
sulfide. The deep-lying Karachaganak gas reservoir field is overlain
by a thick salt formation extending from near the surface in some
places to depths of 4,000 m.
Construction of a gas-condensate
processing plant for the Karachaganak field was begun in 1983.
106,107
Project Lira began on July 7, 1983, with three 13.5-kt explosions at
5-minute intervals. The first two were at a depth of 917 m, and the
last at 841 m. They were followed about a year later on July 21,
1984, by another three 13.5-kt explosions at intervals of 5 minutes.
Their depths of burst were 846 m, 955 m, and 844 m, respectively.
The yields were almost double most of the yields at Astrakhan,
presumably because of the distance of the Lira site from population
centers.
These six Lira explosions would be expected to produce about 300,000
m3 of underground storage capacity for use in conjunction with the
Karachaganak gas-condensate reservoir. At last report, four of the
six, 1T through 4T, were being prepared for use for storage of gas
condensate and partial separation of the natural gas, and one other,
6T, was being held in reserve.
The last one to be fired, 5T,
developed a leak, and the cavity and emplacement hole have filled
with water. As of 1994, plans were being developed for closure of
that location and sealing of the emplacement/access hole.
As with the explosions at Orenburg and Astrakhan, all Lira
explosions were completely contained, and radiation levels are
reported to be at background levels at the Lira site.108
Summary of storage
Overall, the use of nuclear explosions to produce cavities in salt
for the storage of liquid hydrocarbons proved to be a somewhat
successful application with several serious concerns.
In the three
full-scale applications of the technology, over 800,000 m3 of
storage were produced capable of storing some 400,000 tons of
condensate.
The Russians report the possibility of storing gases up
to a pressure of 14MPa (140 atm.). In all cases, the nuclear
explosive emplacement hole was successfully used for post-shot
access and exploitation of the cavities. Although no measurements
have been reported, the Soviets report that there has been no
contamination of the stored condensate product by radioactivity from
the explosions.
However, the loss of six out of twenty-three cavities by leakage of
water into the cavity raises serious questions about the safety and
viability of the application. Water that leaks into a cavity will
begin dissolving salt from the walls and the resolidified mass of
melt at the bottom of the cavity containing the majority of the
radioactive residue from the nuclear explosive.
With time, the walls
may begin to break up, and the water will become contaminated with
soluble radioactive nuclides from the walls, puddle at the bottom of
the cavity, and become hazardous to bring to the surface. Any
product stored in a partially water-filled cavity will tend to
absorb the water and become contaminated by the radioactivity.
Only
experience can define the magnitude of the environmental risk
presented by this sequence of events.
Deep Seismic
Sounding of the Earth
In 1971, the Soviet PNE Program, in cooperation with the Ministry of
Geology, embarked on the most ambitious and far-reaching
application, the use of peaceful nuclear explosions for the seismic
exploration of vast reaches of the Soviet Union.
In the early 1960s,
the Ministry of Geology had been active in carrying out detailed
studies of the Earth's crust and upper mantle in the northern
Eurasian part of the Soviet Union utilizing chemical explosions
spaced a few hundred kilometers apart along a line. These so-called
Deep Seismic Sounding (DSS) fines permitted study of the crustal
structure to depths of 30-40 km over large areas.
During the late
1960s, the Ministry of Geology began a program of experimental
seismic studies, recording the signals from the PNEs carried out for
oil and gas stimulation and for closure of runaway gas wells. Based
on this experience, they developed a program for extending their DSS
program to explore geologies to much greater depths over distances
of a thousand kilometers and for utilizing the much stronger seismic
signals of nuclear explosions (see section B.5 of Appendix B).
Figure 6 is a map of the Soviet Union showing the location of 15 DSS
lines that were carried out over the next 17 years and the location
of the PNEs associated with the DSS program. Section B.5 of Appendix
B provides the data for the DSS explosions and indicates the DSS
lines listed in figure 6 with which they were associated.109-110
Although the signals from PNEs carried out for other purposes and
weapons tests at Semipalatinsk were undoubtedly utilized,111 the
vast majority of data were generated by the 39 PNEs specifically
fired as part of the DSS program. As can be seen in Appendix B, all
of the DSS explosions were carried out at depths of burial of
500-1,000 m, much greater than the minimum depth required for
containment.
Yields varied between 2.3 and 22 kt, with the majority
less than 10 kt.
The seismic lines extended over
distances that ranged from 1,500 to 4,000 km, with three to four
nuclear explosions typically spaced at distances of 500 to 900 km
along those lines. Hundreds of Taiga portable seismometers were
located along each line, many placed by helicopters because of the
remoteness of the regions, at spacing of about 10-20 km. Their
signals were transmitted to central recording stations.
Almost every year from 1971 to 1984, a different DSS fine was
investigated with three or four explosions. The explosions for a
particular line were generally carried out at intervals of 10 to 30
days in the late summer and fall. However, in one line that involved
only two explosions, they were fired about one hour apart.
Shot
times were often late at night or early in the morning to reduce
cultural background noise. In many cases, the nuclear explosions
were augmented by chemical explosions, sometimes delivered as bombs
from aircraft because of the difficult terrain and lack of roads.
In the early 1970s, several DSS lines in the European and Caspian
regions of the Soviet Union were explored, moving from west to east.
Beginning in 1975, exploration of Siberia east of the Urals began
and was the focus of activity for the next ten years.
Relatively few reports have been published describing the results of
these DSS lines and the crustal and mantle structure derived from
them.
The few to have appeared have generally referred to the
nuclear explosions as large industrial explosions.112,113
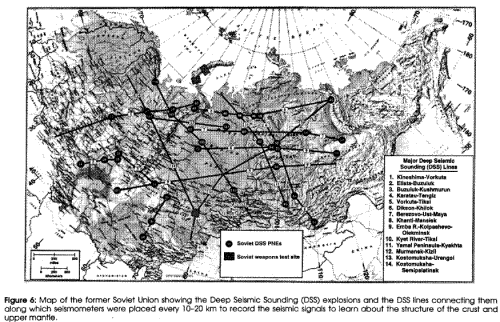
The first publication to include reference to one of the
nuclear lines appeared in 1973,114 but it included little
detail derived from the nuclear explosions.
It was not until 1977-78
that results began appearing in geophysical journals. Generally,
geologic profiles derived from DSS lines utilizing nuclear explosion
sources are characterized by structural details and velocity
profiles to depths of 200-300 km, well below the 35-km depth of the
Morohovic velocity discontinuity, which generally limits the depth
of conventional profiles.
Papers describing the geologic structure
of specific DSS lines utilizing PNEs can be found in endnotes 108
and 109. More recent structural studies can be found in endnotes
113-115-116-117
Much of the data collected on the DSS profiles
has now been made available to other agencies and, through exchange
agreements, with geologists from other countries.118
The limited
results from the use of these data may well be due to the limited
computational capability available to the ministry of Geology who
closely held the data for many years.
Although specific details have not been provided, this work is
described as being of great value not only for defining the general
structure of the crust but also for identifying areas with high
potential for gas, oil, and mineral development. Specifically,
MinAtom claims that the existence of ten gas and gas condensate
deposits in the Yenisey-Khatanga Basin east of Norilsk and about ten
more in the developing areas of the Vilyuysk syncline in Eastern
Siberia have been confirmed through use of data from the DSS
profiles.
All of the DSS explosions were carried out without escape of
radioactivity, with three exceptions. The third and fourth DSS
explosions on the first DSS line in 1971, "Globus-1" and "Globus-2,"
suffered minor leakage of gaseous radionuclides from the emplacement
hole. The areas near the emplacement holes were decontaminated, and
radiation levels are at or near regional background.
A more serious vent accompanied the "Kraton-3" explosion on August
24, 1978, 120 km from the remote village of Aikhal in Siberia. This
is in a region of permafrost. In the process of drilling or stemming
the emplacement hole, some of the medium adjacent to the hole was
melted, which resulted in a failure of the stemming seal and a
dynamic vent of gaseous radioactivity and steam to the atmosphere at
the time of the explosion.
Measurable radioactivity was carried 150
km over unpopulated forest and tundra. Radiation surveys in 1990
showed levels of 1 mR/hr in the immediate vicinity of the hole and
up to 0.2 mR/hr as far as 5 km in the fallout trace.
Following
decontamination of the area, levels are reported to be 30-50
µR/hr
outside a 2 km exclusion zone, which is periodically monitored.119,120
Breakage of
Ore
As experience was gained with
underground nuclear explosions in hard rocks from the nuclear
weapons tests, the idea of using PNEs to assist with mining ores and
other minerals from the earth quickly developed both in the U.S. and
the Soviet Union.
In the late 1960s, the Soviet PNE Program began
looking for a good site to apply this new technique.
The U.S.
Plowshare Program had looked at several techniques for using nuclear
explosions to break up large ore bodies for in-situ processing to
recover the valuable resource. Most of these techniques envisaged
placing a 10-20-kt nuclear explosion at the bottom of a deposit and,
depending on the collapse of the explosion cavity to form a
collapsed chimney of broken rock, above the explosion point.
Recovery would be carried out utilizing drifts mined back through
the chimneys.
Soviet PNE scientists at Chelyabinsk, in cooperation with engineers
from the Moscow Mining Institute and the All-Soviet Institute of
Industrial Technology (VNIPI), developed a concept for using much
smaller explosions (24 kt), which were more suitable for the more
widespread small deposits.121,122
This concept involved mining
vertical slots about 45-60 m/kt1/3 distant from the explosion to
provide a free surface that would reflect the shock wave from the
explosion, greatly enhancing the volume and extent of breakage of
the rock and reducing the compaction.
It was expected that five to
ten times more rock would be fractured in this way than would be
found in the chimney from the same yield explosion. The slot would
also weaken the shock wave and help to protect any other workings or
structures beyond the slot.
A network of crossdrifts would be mined
below the body of broken ore for recovery by the usual stope-mining
methods.123
Project Dnepr
The first experiment using this concept was carried out on September
4, 1972, in the Kuel'por apatite (phosphate) ore deposit about 21 km
north of the city of Kirovsk on the Kola Peninsula, about 150 km
from the Finnish border (see section B.6 of Appendix B).
A 2.1-kt
nuclear explosion was positioned immediately below the ore body,
which was 60-80 m thick and sloping into the mountain at about a 25-
to 35-degree angle. A 50-m-high vertical slot was mined about 50 m
away on the opposite side of the deposit (see figure 7.a). The shock
wave produced a volume of about 100,000 m3 of broken ore.
In an effort to reduce the possibility of contaminating the ore body
with radioactivity from the explosion, "with the aid of a special
device the radioactive products from the explosion cavity were
ejected into barren rock" some 120 m away from the ore body. This
technique was first tested by the Chelyabinsk Laboratory in the
"148/1" test in the Degelen Mountains at the Semipalatinsk Test Site
a year and a half earlier on April 9, 1971.
No information is
available on how well this technique worked, but an additional test
of the idea was carried out at Degelen in the "148/5" event on
December 16, 1974, this time by the Arzamas Laboratory124,125 (see
Appendix C).
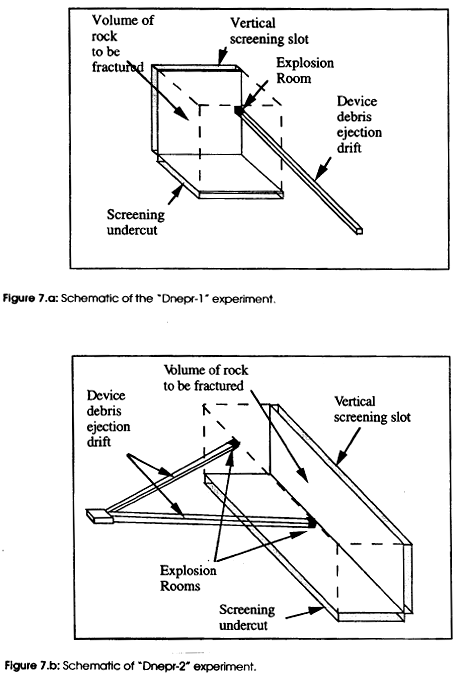
Twelve years later, a second explosion, "Dnepr-2," was carried out
in the mine on August 27,1984.
For this experiment, two 1.8-kt
explosions were fired simultaneously in separate drifts 75 m apart.
The scheme for ejecting the device debris out of the cavity and down
a 120-m tunnel was again utilized to remove radioactivity from the
region of broken ore. Although details have not been provided, the
method of ejecting the radioactive debris from the explosion down a
tunnel and out of the valuable ore deposit is described by MinA-tom
as a success.126
Again, in preparation for this explosion, a vertical screening slot
was constructed about 50 m from the explosions that was 125 m wide
and extended 40 m above the explosion horizon and 50 m below.
Screening drifts were also mined below this block of ore (see figure
7b).
The shock wave from the Dnepr-2 explosions fractured about
500,000 to 600,000 m3 of ore. In total, the two experiments were
calculated to have broken over one and a half million tons of
apatite ore.
A total of 396,000 tons of ore broken by Dnepr-1 and Dnepr-2 were
removed from the Kuel'por mine over the period from 1972 to 1990
using standard stope-mine practices in the drifts below the blocks
broken by the explosions. Secondary blasting required only about
12-13 g of HE per ton to break up oversized blocks instead of the
80-100 g per ton required by the usual mining practice in this
deposit.
Immediately after the Dnepr-1 explosion, leakage of gaseous
radionuclides led to exposures in the city of Kirovsk of 30-40 mR,
about 10 percent of annual background doses, during its passage and
dissipation.
Leakage from the 1984 explosion was delayed by up to 10
hours and led to little exposure off site.
Radiation conditions in the mines were reported to be essentially
the same as in normal mining operations and did not exceed
established safety norms; thus, no special measures were required
for miner safety beyond standard practices. Radioactivity levels in
the ore were also reported to be below permissible levels.
Air and
water within the mine and in the nearby rivers and lakes were
routinely monitored for various radionuclides such as Sr90, Cs137,
Pu239, and tritium. MinAtom reports that no radioactivity levels
above maximum permissible dosage were observed, except for tritium
in water from the ore body, which exceed maximum permissible levels
by a factor of 1.5 to 2 on occasion.
In general, radiation levels in
the area surrounding the site are reported to be at background
levels.127
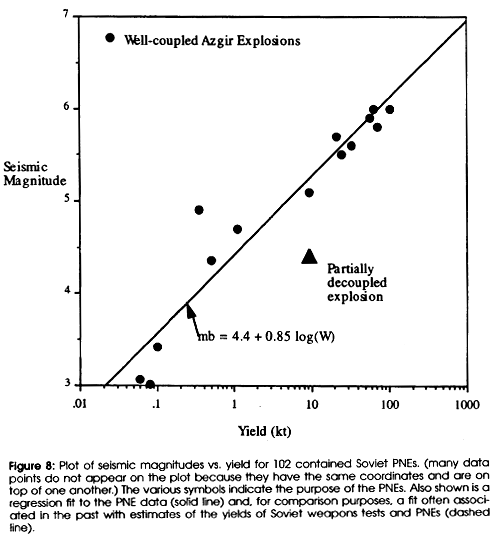
The Dnepr site has been closed since 1992,
but it is subject to
periodic monitoring.
Overall, the experiments are described by MinAtom as being successful, although, as of 1994, the mined ore was
awaiting the construction of an access road before it could be sent
to a refinery for processing.
However, as information about the
Dnepr experiments became public in the late 1980s and early 1990s,
environmental organizations in the area have raised protests and
called for an end to any further such experiments.128
Disposal of
Toxic Waste
Industrial development in the Soviet Union over the last 60 to 70
years has left a legacy of industrial contamination that is probably
unparalleled in the modern world.
Contamination of water supplies by
dumping industrial wastes of chemical and oil industries into rivers
and lakes has endangered the drinking water supplies of many towns
and cities.
In an effort to develop a technology that could be used
to dispose of some of the worst types of industrial effluents and
buy time for the development of better industrial waste treatment
procedures and facilities, early in the 1970s the scientists from
the Soviet PNE Program and the Ministry of the Oil Refining and Oil
Chemical Industries proposed two experiments using nuclear
explosions to produce deep disposal facilities (see section B.7,
Appendix B).
Deep-well disposal utilizes the fact that a 5 to 10-kt nuclear
explosion creates a large fractured region, which, in some cases,
extends to 100-200 m from the explosion, and that the chimney
produced by the collapse of the cavity would contain 100,000 to
150,000 m3 of broken and crushed rock and 30,000 to 50,000 m3 of
void space.
The large central chimney provides surface area and
volume for the deposition of suspended particulates, and the large
fracture radius provides an enormous area for liquids to percolate
into the surrounding formation.
To assure that percolation of the
chemical wastes or any radioactivity leached from the glassy melt
would not contaminate potable water supplies, the geologic layer
must be deep and isolated from any mobile water layers.
Proiect Kama
The sites chosen for the two "Kama" waste-disposal experiments were
not far from the first oil stimulation site (Butane) and the
gas-condensate storage sites (Magistral, Sapphire, and Lira) in the
Bashkir Republic, 30 km west of the city of Sterlitimak (see figure
5).
A depth of burial about 2,000-2,100 m was selected that would
place the explosion and chimney in the middle of a 400-m-thick,
dense carbonate section (dolomite), which was isolated from potable
water sources. Both explosions used special 10 kt devices,
sufficiently small that the size of the emplacement holes were
determined by pumping requirements.
The first Kama explosion, "Kama-2," was fired on October 26, 1973.
Exploitation of the site did not begin until 1976, when industrial
waste began to be pumped from the Sterlitimak soda factory into the
chimney through three holes.
Flow from the soda factory contained
50-100 mg/L of suspended solids that were chemically incompatible
with the water in the injection layer resulting in the precipitation
of more than 1,000 mg/L of additional solids. In a normal injection
hole, this would have rapidly led to plugging of the hole. However,
in the case of Kama-2, more than 23,000,000 m3 of industrial wastes
carrying more than 1,000 tons of suspended solids have been disposed
of in the period between 1976 and 1993.
Use of the "enlarged"
disposal hole continues with an average flow of 4,000-5,000 m3 per
day. Savings to the environment over that same period of time
through the use of, this site have been put at more than 70 million
rubles (1990).
The second Kama explosion, "Kama-1," was fired 10 months later on
July 8, 1974. Exploitation of this site did not begin until 1983
when the disposal of highly toxic waste flows from the Salavat oil
refinery began. This waste contained suspended particulate with
resinous materials having an exceptionally high capability to clog
pores in any conventional disposal site.
These flows contained
between 100 and 1000 mg/L of these particulates and were not
disposable by any other method known at the time. In the period
between 1983 and 1993, about 700,000 m3 of industrial waste from the Salavat oil refinery were disposed at an estimated savings to the
environment of 100 to 200 million rubles (1990).
Observation holes are being used to monitor water tables in the area
for the transport of waste materials and radionuclides from the
sites. To date, no industrial waste material has been detected in
the water layers overlying the injection layer. Observation holes
500-1,000 m from the explosion sites at the depth of the injection
layers detected radioactivity in the water flowing out of the
chimney into the carbonate formation, but within 5 to 6 months after
injection began, the gamma activity level was essentially at
background.
The explosions were completely contained with no prompt vent or
leakage of radioactivity. Although there are some slightly
contaminated areas near the emplacement holes - probably due to
reentry drilling and water table observation holes - beyond the
industrial area the radiation levels are reported to be at
background.129-130
Overall, this would appear to be a highly successful application.
For that reason, it is curious that it was not used at any other
sites in the Soviet Union or Russia in more recent times.
Transplutonic
Element Production
From the beginning, Soviet scientists at the nuclear weapons
laboratories were interested in the possibilities of using the large
flux of neutrons generated in a nuclear explosion for scientific
purposes and to breed new, heavier elements.
Interest in such applications played a
key role early in the U.S. Plowshare Program. Such applications are
the subject of papers at both the. first and second Plowshare
Symposiums in 1957 and 1959 131,132 and of the design of
the first U.S. Plowshare experiment, Project "Gnome."133
As discussed above, interest by the U.S.
in using the high flux of neutrons focused on designing special
nuclear devices to produce new, super-heavy elements well beyond
uranium and plutonium through multiple neutron capture. The ultimate
goal was to determine the physical properties of any new elements
produced and to advance our understanding of the atomic nucleus.
Early on, the interest of Soviet scientists in the use of large
neutron fluxes was directed at the possibility of using them to
produce trace quantities of transplutonic actinide elements through
multiple neutron capture as well as useful quantities of plutonium
238 and 239 and uranium 232 and 233 through single or double neutron
capture. Any such elements produced would then be recovered and used
for isotope production or as fissile material.
Such elements can be produced internal
to a nuclear device as well as by capturing the neutrons produced by
a nuclear explosion in a uranium 238 or thorium 232 blanket placed
around the nuclear device. The difficult problem is recovering the
isotopes in an economical and timely fashion. The Arzamas Laboratory
was the lead laboratory for this program (see section B.8, Appendix
B).134
One of the primary purposes of the first two explosions in the salt
domes at the Azgir site, Halite A-1 and Halite A-2, was to provide
an experimental site for studying the potential of salt cavities for
use in the production of these new isotopes.
A nuclear explosion
detonated in a large water-filled cavity will deposit the vast
majority of its energy in the water. A small portion of the water
would be immediately vaporized, but if the cavity is sufficiently
large, the average temperature of the water in the cavity will be
elevated only a few degrees.
For example, if a 1-kt explosion were
detonated in the Halite A-2 cavity containing 140,000 m3 of water,
it would raise the temperature of the water about 7°C. The water
would then cool and congeal the vaporized debris of the device and
any material close to it.
The condensed debris would then settle to
the bottom of the cavity where it could be easily recovered.
Halite A-2-1 to A-2-6
Beginning in April 1975,
Soviet scientists began carrying out small explosions in the
water-filled Halite A-2 cavity, using nuclear explosives designed to
enhance neutron capture for the production of transplutonic and
other actinide elements.
The first test, A-2-1, was 0.35 kt at a
depth of 583 m, about 14 m above the center of the 32-m-radius
cavity. Access was through the original emplacement hole. It has not
been reported whether attempts were made to recover debris from the
bottom of the cavity for this explosion, but two years transpired
before the next experiment.
The next experiment, A-2-2, was a 0.10-kt explosion carried out in
the A-2 cavity on October 14,1977, which was quickly followed by the
third explosion, A-2-3, an explosion of only 0.01 kt 16 days later
on October 30. Again, no information is available on whether any
recovery was attempted between these two explosions.
The last three transplutonic element experiments were carried out
about two years later. On September 12, 1978, a 0.08-kt explosion
was again detonated in the A-2 cavity. Three months later, on
November 30, a 0.06-kt explosion followed. Two months later, on
January 10, 1979, the last explosion in this series was fired. It
was the largest of the six at 0.5 kt.
All of these explosives were fired at a depth of about 581-585 m,
which would place them about 14 ± 2 m above the center of the
initial cavity. Since the resolidified melt occupies a spherical
segment on the bottom of the cavity about 10 m thick, such a
position for the explosions would place them approximately in the
center of the water volume.
Unfortunately, no further information has been provided by MinAtom
on the details of the experiments, the methods used to recover
debris from the explosions, or to what extent the program was
successful in producing trans-plutonic and other actinide elements.
All of the transplutonic explosions were carried out without
venting. However, recovery operations undoubtedly resulted in some
contamination of the general area around the emplacement hole. The
Halite A-2 cavity has now been closed, and the area has been
decontaminated.
Beyond the working area, radiation
levels are at natural background levels.135
Halite A-4 to A-11
About a year after the first
transplutonic element experiment, the Soviets began to carry out a
series of large-yield explosions in the Eastern Azgir salt dome. The
first of this series, A-4 on July 7, 1976, was a 58-kt explosion at
a depth of 1,000 m, an almost exact duplicate of the A-3 explosion
in 1971.
It was followed a little over a year later on September 9,
1977, by A-5, a 9.3-kt explosion at a depth of 1,503 m.
Over the next two years, the Soviets carried out five more large
yield explosions, A-7 to A-11, in the Eastern Azgir Dome with yields
that varied from 21 to 103 kt at depths that ranged from 630 to
1,500 m.
As with the cavities created for gas-condensate storage, the Soviets
experienced unexpected results in at least one case. The cavity
created by A-9, the largest yield of all the explosions at Azgir,
collapsed at an early time, and the collapse propagated to the
surface. This resulted in the formation of a subsidence crater 500 m
in diameter and 18 m deep. However, there was no release of
radioactivity during or after the collapse.
The other six cavities
initially were sealed with respect to water leakage and stable.
MinAtom has not reported on the purpose for the series of seven
explosions, but there are several possibilities. They could well
have been intended to be a stockpile of cavities for possible use in
future production of fissile materials, using the "breeding" ideas
then being studied in the transplutonic production experiments in
the A-2 cavity.
However, the great variation in the yield and size
of the resultant cavities would be somewhat inconsistent with such
use. In addition, four of these explosions (A-7, A-8, A-10, and A-ll)
were multiples, with two or three explosions in the same emplacement
hole being fired simultaneously.
This would suggest that they were
actually weapons tests diverted from the Semipalatinsk Test Site.
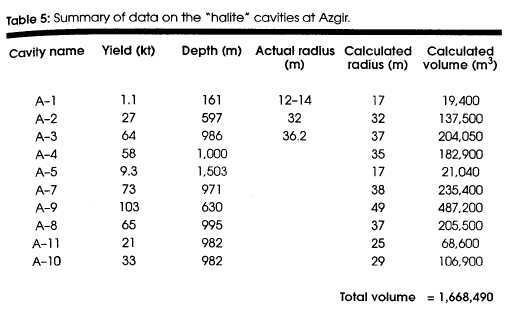
Such a purpose
would also explain why the yields were so variable.136 Finally, they
could be a combination, weapons tests that were used to create
cavities at Azgir for future industrial use.
Table 5 summarizes the data on the Halite cavities at Azgir together
with calculated radii and volumes using the formula developed in the
U.S. Plowshare program.137 Although there is relatively little
agreement for A-l, which was poorly documented because of collapse,
the agreement for A-2 and A-3 is excellent.
Of particular interest is A-5, which had
an order-of-magnitude greater yield than A-l but which, because of
the much greater depth of burial, produced a cavity about the same
size as A-l. The total volume calculated for all the cavities at
Azgir is about 1,670,000 m3, in good agreement with the MinAtom
number of 1,600,000.138 In all, nine standing cavities with
diameters ranging from 34 to 76 m were formed with a total volume of
about 1,200,000 m3, two of which are full of water.
All of the Azgir explosions in this series were completely contained
except for A-8, which experienced early leakage of inert radioactive
gases at 60 min.
Of particular interest is A-5, which had almost an
order-of-magnitude greater yield than the emplacement hole. During
planned reentry into the explosion cavities for post-shot
examination and utilization, a total of 4.7 mCi of radionuclides
were vented to the atmosphere under controlled conditions.
At present, five of the nine standing cavities (A-1, A-2, A-3, A-4,
and A-5) have been flooded by water leaking in from overlying
aquifers.
By late 1990, sites A-1, A-4, A-7, A-8, and A-11 had been
closed and decontaminated. Radiation levels in the areas near the
emplacement/access hole were 8-20
µR/hr, about equal to background.
As of April 1993, sites A-2, A-3, A-5, and A-10 were being closed
and decontaminated, if necessary. The A-10 cavity is being used for
burial of soil from throughout the Azgir complex.
The radiation
conditions of the entire site have been well documented and are
under periodic radiation monitoring.139
Seismic
Decoupling Experiment
Early in the history of test ban discussions between the U.S. and
the Soviet Union, the concept of decoupling or reducing the seismic
signal from a nuclear explosion was introduced.
In the fall of 1958
at the Geneva Conference on Banning Nuclear Tests, the U.S.
submitted the argument that any test ban agreement must take into
consideration the possibility that a nuclear weapon test carried out
inside a large cavity would have its seismic signal reduced by a
factor of 100-300, making it very difficult to detect by seismic
means.140
The Soviet Union very strongly rejected the concept and
refused to consider any of the theoretical arguments made by U.S.
scientists.
In an effort to prove the feasibility of the decoupling concept, the
U.S. Vela Program sponsored a pair of tests in the mid-1960s. In the
1964 SALMON explosion, the U.S. fired a 5.3-kt device at a depth of
828 m in a salt dome near Hattiesburg, Mississippi, to create a
34-m-diameter cavity.141 Two years later, in the STERLING test, a
0.35-kt nuclear explosion was detonated in the SALMON cavity.
The
scaled radius of the cavity was only 24 m/kt1/3. The seismic signal
was partially decoupled by a factor of about 72, confirming the
decoupling concept although not testing the full decoupling
capability of the technique. That would have required a larger
cavity or a smaller-yield decoupling explosion.142
In the spring of 1976, the Soviets decided to carry out a similar
seismic-decoupling experiment in the A-3 cavity (see Section B.9,
Appendix B). On March 29, they fired "Halite A-3-1," a 10-kt nuclear
explosion at a depth of 990 m in the A-3 cavity.143 That depth would
place it approximately at the center of the cavity. The scaled
radius of the cavity was only 16 m/kt11/3 in terms of the decoupled
explosion yield. Because of the smaller scaled radius of the cavity
relative to the STERLING explosion, it was decoupled even less.
Figure 8 is a plot of the seismic body wave magnitudes recorded for
most of the explosions in salt at Azgir, including the transplutonic
production explosions in the water-filled A-2 cavity as well as the
signal from A-3-1, the decoupled explosion in the A-3 cavity. Also
shown is a regression fit to the fully-coupled Azgir data. Based on
calculations by U.S. scientists, the decoupling factor for A-3-1,
using a yield of 10 kt, would be about 15 based on teleseismic data,
and 23 based on close-in data (recorded at less than 100 km).144
The relatively low degree of decoupling produced by this equipment
would contribute little to the issue of how full decoupling could be
used to hide weapons tests under a comprehensive ban on testing,
raising questions as to whether this was the primary purpose of this
test.
An alternative rationale, reported by Vitaly Adushkin,
Director of the Russian Institute for Dynamics of the Geosphere, is
that the purpose of the second explosion in the cavity was for
investigating how much additional volume such a secondary explosion
could produce in a cavity with minimal seismic disruption to the
surrounding territories.
Some of the locations being considered for
storage applications were sufficiently close to populated regions
that the permissible yields were severely limited. In an effort to
produce the required storage volume with a minimum number of seismic
disruptions of the region, the concept of enlarging small cavities
with partially decoupled secondary explosions was under
consideration in the mid-1970s.
Unfortunately, results of this
experiment showed that little additional volume was produced, and no
subsequent application of the idea was made.145
Mine Gas
Dispersal
Coal mining in the Donets Basin of the Ukraine has long suffered
from the dangers of rock bursts and explosions resulting from
pockets of methane gas, which has resulted in the deaths of miners
by explosions and suffocation.
As the coal mines in this area went
to deeper and deeper levels, the problem worsened.
By the end of the
1970s, the number had reached over 200 explosions or rock bursts per
year. At some point in the late 1970s, scientists from the Ukrainian
Academy of Sciences' Fuel Physics Institute and the All-Soviet
Institute of Scientific Industrial Technology suggested using a
relatively small nuclear explosion to open incipient fractures and
equalize stresses in the rock to avoid these sudden and dangerous
occurrences.
A similar effect had been observed in the Sakhalin area
of Siberia as a result of frequent earthquakes in that area (see
section B.10, Appendix B).
Project Cleavage
The site chosen for the
"Cleavage" experiment, sponsored by the Soviet Coal Ministry, was in
the "Young Communist" coal mine 5 km east of the Ukrainian village
of Enakievo.
A 0.3-kt nuclear device was emplaced in the 'Yunkom"
mine in a sloping drift between two of the most dangerous layers, 45
m below a layer named "Devyatka" and 31m above the "Kirpichevka"
layer. The yield was small to minimize damage to the mine and
underground structures as well as the housing in the Yunkom area and
the town of Enak-ievo.
The particular location in a sandstone rock
was chosen to assure that the resolidified melt containing about 95
percent of the radioactivity of the explosion would be insoluble in
water to minimize the transport of radioactivity from the explosion
area. The inclined shaft was sealed with concrete to prevent release
of the gaseous radionuclides to the surface or into other workings
in the mine.
Radiation levels in the air and water within the mine
and at the surface were continuously monitored.
The explosive was fired at 05:00 local time on September 16, 1979,
at a depth of 903 m. Post-shot inspection of the mine revealed
little structural damage beyond the immediate vicinity of the
explosion. Mining in the Devyatka layer in 1980-82 indicated that
the effective radius of the explosion for elimination of the
dangerous blowouts was about 150 m, but there was a significant
reduction of the number and intensity of bursts beyond that distance
as well.
The occurrence of bursts in this time frame are reported to
have been reduced to less than one per million square meters, and
the intensity of any single burst to less than 50 tons, a factor of
4-5 less than that reported earlier.
Radiation levels in the coal mined from the Devyatka layer near the
explosion were reported to be at background levels. Work in the mine
in subsequent years proceeded with no complications from the
explosion, and production increased significantly from 1980 through
1982.146
Unfortunately, the test was carried out without the knowledge of the
local population, and much suspicion of the results remains in the
local area.
Several recent articles in the popular press have cast
doubts on the efficacy of the test, quoting the director of the Yunkom mine,
V. G. Revskovo, as saying there were essentially no
positive results of the explosion in the mine and claiming that the
high residual radiation levels from Chernobyl have made it difficult
to confirm the statements made by the ministry of Atomic Energy
regarding radiation conditions.147-148-149
The fact that in the nine
years following Cleavage, there were no subsequent nuclear
explosions for this purpose in Yuncom or any other mine, would
suggest that the results were less favorable than expected.
Overall
Seismic Coupling
Figure 9 is a plot of the seismic magnitude, determined by the
National Earthquake Information Service or the International Seismic
Center, versus the MinAtom yield for 102 of the Soviet contained
PNEs.
Several of the contained PNEs were not detected by the
world-wide seismic networks because of the small yield or "noise"
from large earthquakes.
The two Dnepr mining shots are also not
included because of their relatively shallow burial. The six
explosions in the water-filled A-2 cavity have been included because
they would have been very well coupled. Also shown is the decoupled
explosion Halite A-3-1 fired in the A-3 cavity.
The solid line in figure 9 is a least-squares regression fit to the
102 well-coupled PNE explosions. The standard error is about 0.29,
corresponding to a factor of about 2.5 in yield. The large scatter
of the data can be attributed to the wide variation in the depths of
burial and types of geology, ranging from porous carbonates to
high-coupling granites and salt.
The dashed line is the
magnitude-yield equation used for many years to determine the yield
of Soviet nuclear explosions from seismic magnitudes.150-151
Virtually all of the Soviet PNEs have yields much less than those
predicted by the dashed curve, some by up to an order of magnitude
or more.
Use of the dashed curve or even one with a constant 0.1 or
0.2 units higher would have consistently overestimate the yield of
most Soviet PNEs.
Epilogue
When President Gorbachov declared a moratorium on nuclear weapons
tests in the fall of 1989, he included a ban on peaceful nuclear
explosions as well, ending this active and ambitious program.
While
the new technology appears to have been warmly accepted and
encouraged by some ministries such as the Geology, Oil and Gas
Production ministries, some of the applications such as toxic waste
disposal were not.
Seismic data from the deep seismic sounding
program continue to be used by the scientists -from the various
geophysical institutions in Russia to better understand the deep
geologic structure of the country. Oil and gas continue to be
produced from most of the fields stimulated by nuclear explosions.
Many of the salt cavities in the Caspian region continue to be used
to store gas condensate for the gas industry. The Kama disposal
sites presumably continue to be used, but there was no interest on
the various chemical production industries to develop other sites
when it was possible to do so.
The apatite mining operations at the
Dnepr sites on the Kola peninsula have been closed since 1992.
Chetek
Early in 1991, news reports began to appear regarding the
establishment of a new private company in Russia called Chetek,
which was proposing to carry out peaceful nuclear explosions on a
commercial basis.
Chetek was reported to have been established by
scientists from Arzamas and to have close ties to the Ministry of
Atomic Energy. One of their most widely promoted proposals was to
use nuclear explosions to destroy the enormous stocks of chemical
weapons (CW) distributed around Russia.152
Their proposal called for mining a number of tunnels off a central
adit at the Novaya Zemlya Test Site, and constructing a room some 20
m high and 80 m long at the end of each tunnel. The chemical
weapons, without disassembly, would be placed in these large
chambers with a 10- to 50-kt nuclear device located in the center of
the mass of chemical weapons.
The high-intensity shock wave from the
nuclear explosion would instantly vaporize and decompose the
chemical weapons - casing, high explosives, chemical agent, and all
- rendering them harmless. The non-condensable gases produced
would be difficult to contain, but they would be relatively harmless
if they escaped to the atmosphere in an area such as Novaya Zemlya.
Chetek argued that one or perhaps two 150-kt explosions, involving
multiple explosions in adjoining tunnels, could destroy the Russian CW stockpile reported to be in the neighborhood of 50,000 tons.153
While such a proposal would have a significant economic and time
advantage over conventional methods of CW destruction, particularly
since Russia reportedly does not have an operational CW destruction
facility, some concerns have been expressed regarding this proposal.
The primary concerns center on the consequences of an accident
before or during the nuclear explosions.
While the remote nature of
the Novaya Zemlya site would be a significant advantage, it would
also pose difficult and dangerous problems for the shipping and
storage of chemical weapons. The accumulation of such a large mass
of chemical weapons, including their high explosive detonators, in
one location would represent a terrifying potential for even a minor
accidental explosion.
In addition, while it would could be assured
with a high degree of confidence that the nuclear explosions would
completely decompose the chemical weapons, any misfire in which the
yield was significantly lower that expected could lead to release of
enormous quantities of CW toxins.
In an effort to move their proposals forward, Chetek proposed a
small demonstration explosion on Novaya Zemlya in the spring of
1992, involving the destruction of 20 tons of chemical weapons.
However, they were apparently unable to gain approval of the new
Yeltsin government for suspension of the moratorium on nuclear tests
and the experiment was never carried out.
Although Chetek continued
to seek support for their proposals for the next year or so, by the
end of 1993, Chetek appears to have disappeared.
High-Level Radioactive Waste Disposal
More recently, several proposals have surfaced within Russia
regarding a similar idea for the disposal of high-level radioactive
waste from nuclear power reactors and naval propulsion reactors.
Scientists from Arzamas and the Defense Ministry's Central Institute
of Physics and Technology have suggested placing fuel rods and other
highly contaminated components in a relatively small room
surrounding a nuclear explosive device, again in a tunnel at Novaya
Zemlya. In this case, the room would be limited in size to assure
that all of the radioactive materials would be vaporized and melted,
along with enough rock to ensure entrapment of the radioactive waste
in the resolidified rock melt.
Such burial would ensure isolation of
such radioactive waste from the biosphere for 10,000 years or
longer. Using such a method, a 100-kt explosion could be expected to
permanently dispose of up to 200 tons of radioactive waste at an
estimated cost of about $20 million.154
While both these proposals may have merit from a technical viewpoint
and would help to solve several serious national problems facing
Russia, there appears to be little resolve to overcome the many
formidable political problems, including the recently signed
Comprehensive Test Ban Treaty, which prohibits all nuclear
explosions, including peaceful nuclear explosions.
ARMS CONTROL ASPECTS OF
THE PEACEFUL USES OF NUCLEAR
EXPLOSIONS
Conference on the
Discontinuance of Nuclear Weapons Tests
Almost from the beginning, the concept of using nuclear explosions
for peaceful purposes was recognized as an impediment to the
achievement of a ban on the development and testing of nuclear
weapons.
Early in the 1958 Geneva Conference on the Discontinuance
of Nuclear Weapons Tests, U.S. Ambassador James Wadsworth noted,
inter alia, the U.S. interest in retaining the capability to carry
out peaceful nuclear explosions under a ban on nuclear weapons
tests.
In a paper on the basic provisions for a control regime,
Ambassador Wadsworth included the provision that one of the
responsibilities of the Control Commission would be to authorize PNEs subject to unspecified inspection and control requirements. The
Soviets did not respond, at least initially, to this U.S.
suggestion.
The fundamental problem posed by permitting PNEs to be carried out
under a ban on all testing of nuclear weapons devices is how to
prevent nuclear explosions carried out for peaceful purposes from
contributing knowledge useful to the development of nuclear weapons.
Whereas PNE and weapon devices could well have different design
requirements in terms of size, weight, radiation output, and
residual radioactivity, learning how to design better PNE devices
would directly contribute to designing better weapons.
Development
of "cleaner" explosives with much lower fission-to-fusion ratios
were essential for nuclear excavation applications.
How could the
side conducting PNEs be prevented from testing new device design
ideas, with or without diagnostic measurements of device
performance, even if such improvements were prohibited?
The final
yield or radiochemical analysis of microscopic particles of the
debris from a PNE explosive could provide sufficient proof of the
validity of many new ideas, but only to those who designed the
device.
Initial ideas within the weapons laboratories for how these problems
might be handled included four ideas:
-
Using whatever device a country
desired for the PNE, under observation by representatives of
the U.N. and other countries, including the Soviet Union,
but without diagnostics to measure the device performance.
-
Establishing an international
stockpile with each country desiring to conduct PNEs,
placing some number of devices in the stockpile on the date
a test ban went into effect.
-
Using only devices provided by
the Soviet Union for PNEs conducted by the U.S. and the
U.K., and vice versa.
-
Using devices that were subject
to inspection by all the nuclear weapons states party to the
test ban, including the U.S.S.R.
The first idea would obviously have been
the best for those interested only in PNE applications, but it had
the major difficulty of how to convince the other parties that no
militarily useful information would be gained absent obvious
diagnostic measurements.
On the other hand, it was recognized
that the other three ideas would most probably lead to the use of
obsolete devices for Plowshare projects without any prospect of
using low-fission excavation explosives yet to be developed.155
The USAEC ultimately recommended the international stockpile option to
the interagency group developing the U.S. negotiating position.156
When the U.S. tabled specific language
in December, 1958, that called on the future Control Commission to,
"establish procedures... for the surveillance of nuclear devices and
observation of nuclear detonations for peaceful purposes,"157 the
Soviet representative Semyon Tsarapkin immediately took issue,
arguing that "the purpose of our Conference is to work out a treaty
on the cessation of nuclear weapons tests everywhere and for all
time, and to adopt the necessary measures to ensure that the parties
to the treaty comply with it. Our contention is that no nuclear
detonations should be set off for any purpose whatsoever."158
This blanket Soviet opposition to any testing for any purpose under
a test ban was rather short-lived when 10 days later, on December
25, in a speech to the Supreme Soviet, Foreign Minister Andrei
Gromyko said it might be possible to have nuclear detonations for
peaceful purposes under a test ban if, among other conditions, there
would be an equal number of such shots between East and West and if
all the devices to be used were subject to complete internal and
external examinations.159
Nine months earlier, Edward Teller had
made a similar suggestion in testimony before Congress that,
"in
order to have an effective international inspection (of peaceful
nuclear explosions) it is necessary not only to have the explosion
inspected, but to open up the explosive, look into it and see that
it is an ordinary type of nuclear explosive. This could be done, but
it certainly would give away a lot of information which at present
is kept very closely guarded."160
This statement was part of a long
list of reasons why he believed a test ban was a bad idea, a motive
that may well have been the reason the Soviets picked up on the same
idea.
Perhaps heartened by this apparent change in the Soviet point of
view, on January 30, 1959, Ambassador Wadsworth presented a draft
article containing suggested procedures for carrying out PNEs based
roughly on the second of the four ideas listed above.
Four months before the PNE explosion,
the party proposing the test would be required to provide a
description of the project, including the purpose, the date and
location, the expected yield, the measurements and experiments to be
carried out in conjunction with the explosion, and,
"the measures taken to assure that
there will be no substantial fall-out outside the immediate
vicinity."161
Further, the U.S. proposal called for
the establishment of a depository, under the surveillance of the
Control Commission, in which any of the parties to the agreement,
prior to the effective date of the agreement, could place a
stockpile of nuclear explosives planned for use in their PNEs.
To meet concerns about safety and
reliability, it provided for checks by the depositing party of any
devices in the depository under the watchful eye of the other
parties.
As an alternative to this procedure, the U.S. proposed that new
nuclear devices could be used at any time so long as the other
parties could inspect,
"the internal and external features of the
nuclear device, including... detailed drawings."
Presumably this
provision was aimed at permitting the U.S. to carry out nuclear
excavation projects with new devices with significantly lower
fission yields than those available in 1959. As noted above,
development of such devices was one of the principle elements of the
U.S. Plowshare Program.
Wadsworth argued that the end result of the provisions on the
devices to be used for PNE projects would,
"be to ensure either that devices
based on past technology will be used, thus foreclosing the
possibility of advancing weapons' design by the detonation, or
that the other... parties will be given detailed knowledge of the
device, thus assuring there will be no military advantage to the
party detonating. The first method requires the use of devices
which have been developed before the agreement to discontinue
nuclear weapons testing goes into effect..."
and,
"the second method... is intended to
confine the devices used under that option to relatively
obsolete designs and to designs especially developed which could
be, perhaps because of weight or bulk, not useful militarily and
which could, therefore, be revealed to the other nuclear powers,
but would allow the non-military purposes of such explosions to
be carried on more efficiently and more cheaply."162
Three weeks later on February 23, 1959,
Ambassador Tsarapkin made a more formal reply to the U.S. proposal.
Leading off with a condemnation of any
proposal to permit PNEs under a test ban, he noted that their,
"position is clear that the direct
and only task of this Conference is to prepare a treaty on the
cessation of all types of nuclear weapons tests forever. We
cannot agree with the attempt of the United States delegation to
transform this Conference on the discontinuance of tests into a
conference on the legalization, in one form or another, of the
continuation of nuclear tests."163
However opposed the Soviet Union was to
including PNEs in the test ban, Tsarapkin paradoxically went on to
say that the Soviets were nevertheless willing to allow them, but
under their own set of conditions, which he then placed on the
table.
The conditions provided for equal
numbers of PNEs between the U.S. and U.K. on the one hand and the
Soviet Union on the other. Such a procedure would have effectively
given the Soviet Union veto power over any Western PNE projects and
was clearly unacceptable.
Rather than creating a stockpile of PNE explosives, Tsarapkin
proposed:
Little was said by the U.S. in response
to this Soviet proposal at the time, although the requirements for
internal inspection and turning the blueprints of U.S. devices over
to the Soviets for their perusal would have, at first hand, appeared
to be out of the question.
Nevertheless, the U.S. accepted the
proposal for the time being, and later that year announced at the
U.N. that,
"agreement in principle has been reached that nuclear
explosions for peaceful purposes will be allowed...under carefully
prescribed conditions under international observation."165
During
the ensuing two years, most of the discussion at the Conference was
directed toward the development of a joint program of seismic
research using nuclear explosions, to be carried out under the
existing moratorium.
However, each country's position on where the
nuclear explosives to be used would come from was based on their
previous positions regarding PNE explosives.
Finally, on March 21,
1961, the U.S. accepted the Soviet position providing for full
disclosure and inspection of devices to be used for seismic
experiments as well as for PNEs.166
Little more was done on the development of an accommodation for PNEs
during the Geneva Conference before it ended in January 1962,
following the August 1961 Soviet decision to end their moratorium
and resume nuclear weapons testing, or in the U.N. Committee on
Disarmament which followed.
Limited Test
Ban Treaty (Moscow Treaty)
Eighteen months later in July 1963, ad hoc negotiations in Moscow
resulted in the Limited Test Ban Treaty (LTBT) (or Moscow Treaty),
which banned,
"any nuclear weapon test explosion, or any other
explosion, at any place under its jurisdiction or control:...if such
explosion causes radioactive debris to be present outside the
territorial limits of the State under whose jurisdiction or control
such explosion is conducted...."
The initial U.S. draft had
contained a provision to allow PNEs in the prohibited environments
if there was unanimous agreement and if they were carried out in
accordance with provisions of an annex which had not yet been
drafted, but presumably along the lines of what the Soviets had
suggested at earlier Geneva discussions.167
However, the Soviets
demurred, and the provision was dropped.
The construction of the above article clearly was intended to apply
to nuclear explosions for peaceful purposes as well as for weapons.
However, the choice of words,
"...radioactive debris to be present
outside the territorial limits..." were interpreted by the AEC as
allowing a nuclear cratering explosion so long as it did not result
in "a quantity of radioactive debris delivered outside the country's
territorial limits in amounts sufficient to establish that such
contamination resulted from a recent test within that country."168
All of the U.S. nuclear crater experiments after 1963 were carried
out within this interpretation of the LTBT, although AEC Chairman
Seaborg made the point during ratification testimony that it would
be impossible to carry out a major project, such as excavating a new
Trans-Isthmian Canal in a small country, without renegotiating the
treaty.
As previously noted in the section entitled, "Kama-Pechora
Canal Project," and in endnote 47, the Russian language version of
this particular section of the treaty is much more permissive than
the English language version, although probably not sufficient to
permit major projects such as the Kama-Pechora Canal to be carried
out.
Concerns about possible violations of the LTBT, if it were to carry
out the Kama-Pechora Canal project, led the Soviet Union to
participate in a series of bilateral technical meetings on PNEs with
the U.S. during 1969-76.
In these meetings, they hoped to reach a
joint understanding with the U.S. for interpretation or amendment of
the LTBT language, which would allow large-scale nuclear excavation
projects to be carried out under appropriate radiation safety
guidelines, rather than the "detection-level" standard of the LTBT.
Although several concepts were discussed, there was no resolution of
the issue.169
Non-Proliferation Treaty (NPT)
Later in the 1960s, at the time of the negotiation of the
Non-Proliferation Treaty, the Plowshare Program was still under
active development in the U.S. as was the Soviet "Program for the
Use of Nuclear Explosions in the National Economy," although at a
less developed stage.
This treaty provides for the non-nuclear
weapons states of the world to forego any effort to acquire nuclear
weapons and the nuclear weapons states not to transfer any weapons,
materials, or technology useful for weapons to the non-nuclear
weapons states. In exchange for the non-nuclear weapons states giving
up the right to acquire nuclear weapons, the NPT committed the
nuclear weapons states to make available the peaceful uses of
nuclear uses of nuclear energy - the materials and the technological
information - under appropriate safeguards through the International
Atomic Energy Agency (IAEA).
In an effort to meet demands and build support for the NPT among
some of the more reluctant non-nuclear weapons states (e.g., India,
Argentina, and Brazil), the U.S. suggested, and the Soviet Union
readily supported, the inclusion of a provision, Article V, which
explicitly committed the nuclear weapons states to make available
the,
"potential benefits from any peaceful applications of nuclear
explosions...on a non-discriminatory basis...pursuant to a special
international agreement..." or "...pursuant to bilateral
agreements."
Although several international projects were
subsequently studied by the U.S. jointly with other countries, no
international projects were ever carried out.170
Article V of the NPT did lead to the establishment of an office within the IAEA for
coordinating international interests in peaceful uses of nuclear
explosions. It also led to five international panels or technical
meetings on PNEs between 1970 and 1976, which provided a forum for
technical interaction of scientists from the U.S., the Soviet Union,
and other countries.171
One of the participants in the IAEA Technical Panels on PNEs in the
early 1970s was the state of India, which expressed an interest in
one particular application, the use of nuclear explosions in the
mining of non-ferrous metals
in general and copper in particular.172
On May 18, 1974, India
carried out a "peaceful nuclear explosion experiment" in the
Rajasthan Desert in western India, describing it,
"as a step towards
studying fracturing effects in rocks, ground motion, containment of
radioactivity and the problems involved in access of the
shot-environment."
The explosion triggered a storm of vehement
protests from around the world, rejecting the claimed peaceful
purpose for the explosion and decrying the attempt by India to
establish itself as a nuclear weapons state.
Up until the end of 1995, there was no evidence of any additional
activity by India towards pursuing their professed interest in
peaceful nuclear explosions. Since that time, however, there have
been sporadic reports of preparations for an additional nuclear
test, but to date, there have been none.173,174
The Indian explosion
is perhaps the most clear demonstration of the conflict between
efforts to limit the spread of nuclear weapons technology and
desires to acquire the technology required for peaceful nuclear
explosions.
Threshold Test
Ban (TTB) and the Peaceful Nuclear Explosion Treaties (PNET)
The conflict between the efforts to achieve an arms control
agreement and the potential promise of peaceful nuclear explosions
next arose in the negotiations of the Threshold Test Ban Treaty in
June 1973.
By this date, the Plowshare Program in the U.S. was
rapidly fading, while its star was just beginning its ascent in the
Soviet Union. The roles of the two countries regarding the
desirability of an exemption for PNEs was beginning to be reversed.
The U.S. adamantly supported the position that any yield limit on
nuclear weapons tests should equally apply to PNEs, whether they
were fired on the weapons test site or not. The Soviet Union was
represented by Igor Morokhov, the First Deputy Chairman of the State
Committee for the Utilization of Atomic Energy and one of the
leading advocates for PNEs in the Soviet Union.
Equally adamantly,
he supported the Soviet position that the yields of PNEs away from
the test sites should not be held to the same limit as weapons, or
else the limit should be placed high enough (400-600 kt) that PNE
projects such as the Kama-Pechora Canal could be done. In the end,
it was agreed a 150-kt limit would be imposed on all tests at the
declared weapons test sites, and a separate PNE agreement would be
negotiated over the next 18 months before the TTBT went into effect.
The subsequent PNE agreement was to provide for extensive exchange
of information on geology and other details regarding forthcoming
PNEs and, for the first time in any U.S. arms control agreement, was
to include on-site observation of PNE activities by the other party.
Negotiation of the PNE Treaty required the full 18 months and
resulted in one of the most detailed agreements for the exchange of
information and conduct of on-site observations in the history of
arms control to that date. The PNET, signed in May 1976, provided
for a single device yield of 150 kt but permitted simultaneous group
explosions with a total yield of up to 1,500 kt.
For any explosion
with an aggregate yield greater than 150 kt, the other side would be
allowed to place sensors in holes near the explosion, which would
allow them to measure the yield of individual explosions in the
group.
Although the TTB and PNET were not ratified, both sides agreed to
observe the limitations pending ratification. However, by the time
the PNET was signed in May of 1976, the U.S. Plowshare Program was
dead, and the Soviet Union's interest in the Kama-Pechora Canal
project had waned.
As a result, neither side has ever carried out a PNE with a yield large enough to trigger the on-site provisions of
the PNET, The Protocols to the TTBT and PNET were subsequently
renegotiated in the late 1980s to tighten the verification
provisions, requiring on-site inspection of any PNE with an
aggregate yield greater than 35 kt and on-site hydrodynamic yield
measurement for any PNE with an aggregate yield greater than 50 kt.
The revised TTBT and PNET were ratified in the fall of 1990, but
neither party has carried out a weapons test or PNE that was subject
to the provisions of the treaties since they went into effect.
Comprehensive
Test Ban Treaty Negotiations (CTBT), 1977-80
In the fall of 1976, the U.S. and the U.S.S.R. once again began
negotiations of a comprehensive ban on all nuclear weapons tests,
this time with the participation of the U.K.
The issue of PNEs very
quickly became one of the principal issues with the Soviet Union,
again represented by Igor Morokhov, strongly pushing for some kind
of separate agreement that would permit PNEs. This time, the U.S.,
with U.K. support, was adamant in refusing to consider any such
agreement, and the issue became one of the major sticking points in
the negotiations.
After several weeks of serious discussion of the issue, it became
apparent that a major decision regarding the PNE issue was being
developed on the Soviet side.
One night, in informal conversations
outside the negotiations, Morokhov and his deputy on the delegation,
Roland Timerbaev, asked several members of the U.S. delegation to
consider three possible alternatives for carrying out PNEs under a
CTBT:
-
The Soviet Union would use U.S.
devices for their PNE projects, and vice versa
-
The U.S. would have complete
access to the design of any devices used by the Soviet Union
in a PNE, and vice versa
-
The U.S. and the Soviet Union
would undertake a joint program of developing and
manufacturing the devices to be used in any PNE
The first two proposals were similar to
those discussed in 1958-59 at the Geneva Conference, but the last
one was an idea that would have been unthinkable at that time.
The
U.S. delegation gave little attention to Morokhov's overture. Within
two weeks, on November 2, 1977, Soviet General Secretary Brezhnev
announced that the Soviet Union would accept a moratorium on all
PNEs for the duration of the CTBT. Shortly thereafter, Morokhov was
replaced as head of the Soviet Delegation by Andronik Petros'yants,
Morokhov's superior as Chairman of the State Committee for the
Utilization of Atomic Energy.
Although the PNE issue had been
resolved, other issues led to a stalemate in the negotiations before
they were abandoned by the Reagan Administration in 1981.
CTBT
Negotiations, 1994-96
On October 19, 1989, the Soviet Union began an unannounced year-long
moratorium on nuclear weapons tests.
As with an earlier announced
18-month moratorium on weapons testing during 1985-86, the Soviet
Union carried out no PNEs during this moratorium. Although the
Soviet Union carried out one last weapons test in October of 1990,
no additional PNEs have been fired since September 6,1988.
Following several extensions of the Soviet Union's moratorium,
testing limitations imposed by the U.S. Congress, and a French
moratorium, negotiations among the five nuclear weapons states on a
Comprehensive Test Ban Treaty were begun in January 1994 in Geneva.
As in earlier negotiations, the PNEs immediately became a
significant issue.
From the beginning of these negotiations, the
U.S. and U. K. advocated a ban on all nuclear explosions, including
any for "peaceful purposes."
France, which studied the potential of PNEs for use in and around their country in the early 1970s, also
supported a ban on PNEs. Although China had never evinced any
previous interest in the use of nuclear explosions for peaceful
purposes, it began the negotiations with a demand for inclusion of a
provision that would permit PNEs at some future date.
Russia took
the position of acquiescing in their elimination, but not actively
advocating it.
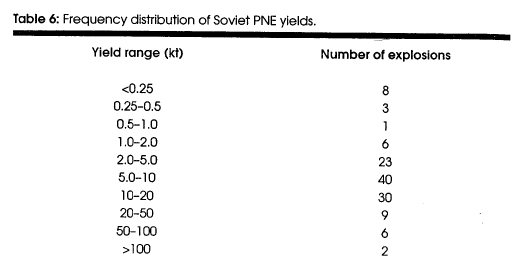
All of the nuclear weapons states maintained
their initial positions on PNEs throughout 1994 and 1995, but on
June 6, 1996, China yielded to the growing pressure for an agreement
and dropped its insistence on an exclusion for PNEs in the
comprehensive test ban agreement, proposing instead that the
question of PNEs should be reconsidered at a review conference,
expected to be held in 10 years.
As a result, final agreement on a CTBT-was quickly reached among all the delegations, with the
exception of India, Pakistan and North Korea. It was signed by all
five declared nuclear weapons states on September 24, 1996.
SUMMARY
During a period of some 23 years between 1965 and 1988, the Soviet
Union's "Program for the Utilization of Nuclear Explosions in the
National Economy" carried out 122 nuclear explosions to study and
put into industrial use some 13 applications.
In all, 128 explosives
with yields ranging from 0.01 to 140 kt were used, with the vast
majority being between 2 and 20 kt (see table 6).
Most peaceful applications of nuclear explosions in the Soviet PNE
Program were explored in depth with a number of tests, but
unfortunately little has been reported on the technical results
other than general outcomes. Two applications, deep seismic sounding
of the Earth's crust and upper mantle and the creation of
underground cavities in salt for the storage of gas condensate,
found widespread use, representing over 50 percent of all the
explosions.
Explosions to explore the technical possibilities of
stimulating the production of oil and gas reservoirs accounted for
an additional 17 percent.
The deep seismic sounding program produced an enormous volume of
seismic data that still are being analyzed to better understand the
deep geologic structure of the vast reaches of the Russian
subcontinent. Although it may assist in the discovery of a few new
major hydrocarbon or mineral resources in the future, its main value
will probably be in the geotectonic area.
The two main projects to create underground storage for gas
condensate from newly developing gas fields appear to be a quite
valuable resource for the industries involved, and may have
expedited their development. However, the failure of almost half of
the explosion cavities created in salt at the Vega and Azgir sites
raises serious questions about the general applicability and
long-term viability of this or any other application utilizing such
cavities.
Clearly, the leakage of water into salt cavities is a
serious problem because of possible loss of the cavity as well as
the leachability of radionuclides trapped in the fused salt and the
surface contamination that will inevitably result.
The studies of oil and gas stimulation were much broader and
longer-term than those carried out in the U.S. The results reported
to date are quite favorable in terms of increased production versus
costs, but the application was not used on an industrial scale in
the Soviet Union.
The reason may be due to the contamination
problems encountered at the Grifon field, but, more likely, it is
because of the same difficulties experienced in the U.S. Plowshare
program - the necessity of large-scale utilization for a
significant impact on the national oil or gas industry, and the
resistance of the public to accept a product containing any added
radioactivity, no matter how minimal the level.
The use of nuclear explosions for closure of four runaway gas wells
that defied all other techniques available in the Soviet Union
proved to be a valuable application.
It is possible that these wells
could have been closed with conventional techniques available in the
U.S., but the experience of the Soviet PNE Program in this area is
unique and may prove useful in some future emergency somewhere in
the world. It is important that Russians make available more
information on why the attempt at Nar'yan Mar failed.
The Dnepr ore-breakage experiments on the Kola peninsula appeared to
have been successful, but the lack of implementation on a broader
scale raises questions about the acceptability of the application.
Similarly, the Cleavage mine gas-dispersal explosion would appear to
be an application with limited applicability, significant problems
of acceptability, and little support within the industry.
The use of nuclear explosions to produce actinide and transplutonic
elements in water-filled cavities is an interesting and imaginative
application, but it is difficult to imagine that such a procedure
could produce significant quantities of such nuclides in comparison
to nuclear reactors. On the other hand, such an approach might prove
quite useful in a heavy-element production program similar to that
carried out by the U.S. in the 1960s to produce super-heavy elements
beyond element 110.175
Many of the heavy elements produced by
multiple neutron capture within a nuclear explosive have very short
half-lives.
Through the use of this technique, significant
quantities of transplutonic elements produced in an initial
heavy-element explosive could be rapidly recovered from a cavity,
processed, and incorporated as target nuclei in a second
heavy-element explosion. Similarly, any super-heavy elements
produced in the second explosion could be rapidly recovered and
processed, maximizing the possibility of detecting any short-lived
isotopes.
One of the most useful applications, from the standpoint of Russia
and other countries with serious environmental contamination
problems, would appear to be the Kama experiments demonstrating the
use of deeply buried nuclear explosions for the deep-well disposal
of toxic industrial flows.
Results from these two experiments
indicate that such facilities can be used for the disposal of large
quantities of very hazardous chemical pollutants over long periods
without significant problems. In view of the apparent success of
these experiments, it is difficult to see why there have been no
subsequent use of this application at other sites.
The nuclear excavation element of the Soviet PNE Program proved to
be relatively short-lived, suffering from the world-wide growth of
public concerns about environmental issues and atmospheric
radioactivity and the disappointing Taiga experiment. In addition,
the Soviets had serious concerns about dealing with the restrictions
of the Limited Test Ban Treaty on the release of radioactivity from
nuclear explosions across national boundaries, particularly for a
project the size of the Kama-Pechora Canal.
In the early 1970s,
environmental concerns about diversion of water from the Arctic
Ocean led to the loss of governmental support for the Kama-Pechora
Canal. In addition, the slumping of the Taiga cratering experiment
in 1971 demonstrated that the geology along much of the alignment of
the canal was not suitable for nuclear excavation.
Although the
project continued to be included in future plans for several years,
it ultimately was dropped, and with it, any further support for the
Soviet nuclear excavation program.
An important element in the Soviet PNE Program was the effort by
both the Arzamas and Chelyabinsk weapons laboratories to develop
special nuclear explosives designs to reduce the radioactivities and
fielding costs associated with specific applications (see Appendix
C). The earliest effort was the development of small-diameter,
high-temperature explosives for use in the closure of runaway gas
wells.
At the same time in the late 1960s and early 1970s, a major
effort was put into developing a very low-fission explosive for
nuclear excavation projects. Unfortunately, the Russians have not
provided any details on the extent to which they succeeded in
meeting their objectives in these device development programs.
In 1971 and 1974, the Soviets carried out two experiments at the
test site to explore the possibilities for ejecting the
radioactivity from an explosion down a tunnel to separate it from
the physical effects of the explosion.
The results of these tests
were subsequently used in the Dnepr 1 and 2 mining experiments.
These tests were similar in geometry to the 1967 Marvel test in the
U.S. Plowshare Program, although the purpose of Marvel was to drive
hydrodynamic energy, not radioactivity, 100 m down a 1-m-diameter
tube.
In the mid-1970s, emphasis in the Soviet device development
program presumably was on the development of small-diameter,
low-tritium-producing explosives for hydrocarbon applications, such
as oil and gas stimulation and gas condensate storage. MinAtom says
this program was successful in meeting its goals, but it provides no
details.176
The Soviet programs to develop low-fission excavation and
hydrocarbon explosives mirrored device development programs in the
U.S. Plowshare Program in the mid-1960s and early 1970s. The
Russians have not provided any information on whether the devices
used in the transplutonic element production program were specially
designed for that purpose as were the devices used in the U.S. heavy
element program.
With the exception of the cratering explosions at the test site and
at Taiga on the Kama-Pechora canal alignment, the vast majority of
the other 112 Soviet PNEs were completely contained (camouflet)
explosions. Five resulted in the prompt release of radioactivity,
the most serious of which was Kraton-3, a DSS explosion in Siberia,
which suffered a prompt vent of gas and particulate radioactivity
when the permafrost around the emplacement hole melted.
The Crystal retard dam in Siberia also resulted in the escape of gaseous
radioactivity, as had been expected in the design of the experiment.
Three other explosions, Globus-1, Globus-3, and Halite A-8, suffered
leaks of gaseous radionuclides at early times as a result of leaks
in the emplacement hole stemming.
In all, there was escape of
gaseous radioactivity at 26 sites, largely during operations to
re-enter the cavities or chimneys or during industrial exploitation
of the sites. In all cases of camouflet explosions, except Kraton-3
and Crystal, the sites have been decontaminated, and radiation
levels outside the limited industrial areas are at regional
background.
The Soviet PNE Program was conducted as a "secret" program in the
Soviet Union, as were many other governmental operations during that
time. Although a few articles appeared in the popular press in the
1970s, there were no details on the number or location of
explosions. As a result, local populations were seldom informed of
the nature or scope of PNE activities in their vicinity.
With the
arrival of Glasnost in the late 1980s, many "exposes" began to
appear in Russian newspapers and journals listing the many PNEs that
had been carried out throughout the country and, in many cases,
viewing with alarm their consequences. Because of the generally low
standards of industrial safety and environmental protection in the
Soviet Union and Russia, the public is quite prepared to believe the
worst.
The lack of hard data from MinAtom on the good and bad
results of their PNE experiments has made it difficult to develop a
rational discussion of their costs, risks, and benefits.
The Soviet PNE Program was many times larger than the U.S. Plowshare
Program in terms of both the number of applications explored with
field experiments and the extent to which they were introduced into
industrial use. Several PNE applications, such as deep seismic
sounding and oil stimulation, have been explored in depth and appear
to have had a positive cost benefit at minimal public risk.
Several
others, such as storage, developed significant technical problems
that cast a shadow on their general applicability. Some, such as
closure of runaway gas wells, demonstrated a unique technology that
may yet find application in a situation where all other techniques
fail. Still others were the subject of one or two tests but were not
explored further for reasons that have not been explained.
Overall,
the program represents a significant technical effort to explore a
promising new technology, and it generated a large body of data that
appears to be quite favorable, although only a small fraction of the
data has been made public.
However, the fundamental problem with PNEs - first identified by
James Schlesinger shortly after he became Commissioner of the U.S.
AEC in 1971 - is the fact that, if they are to be economically
significant, there must be widespread use of the technology, and
such use must inevitably involve large numbers of sites, each of
which presents a potential source of radioactivity to the
environment in general and to nearby communities in particular.
Russia now has more than 100 sites where a significant amount of
high-level radioactivity has been buried, albeit at a deep, safe
environment.
However, activities at these sites must be restricted
and monitored forever. Even though each site can be operated well
within appropriate radiation-safety standards, and the industrial
products exported from the sites may be many times below maximum
permissible levels, experience over the last 20 years in the U.S.
and in today's Russia shows that it is virtually impossible to gain
public acceptance of such applications of nuclear energy.
In addition to the problems of political and economic acceptability,
PNEs also pose a difficult problem in the arms-control arena in the
context of a total ban on nuclear weapons tests. In the absence of
any other form of nuclear testing, any nuclear explosion for
peaceful purposes has the potential for providing useful information
to those who designed and constructed the nuclear device.
Thus,
under a CTB, any country conducting PNEs would, in appearance if not
in fact, receive information useful for designing new nuclear
weapons or maintaining an existing nuclear stockpile, information
denied to the other parties to the treaty.
Although several
imaginative ideas for reducing this risk have been offered in the
course of negotiations on a CTBT over the last 40 years, none have
been suggested that would appear to overcome this critical problem.
ACKNOWLEDGMENTS
NOTES AND
REFERENCES
1. United Nations General Assembly,
Official Records: Fourth Session, Ad Hoc Political Committee,
Thirty-Third Meeting, (November 10,1949), p. 188.
2. Pokrovskiy, G. I. "Beginning of an Era of Atomic Energy,"
Tekhnika Molodezhi,Vol. 9, (1954).
3. Reines, Frederick, "Are There Peaceful Engineering Uses of
Atomic Explosives?," Bulletin of Atomic Scientists, (June 1950),
pp. 171-2.
4. Goldschmidt, Bertrand, The Atomic Complex, American Nuclear
Society, (1982), pp. 257-62.
5. Rougeron, Camille, Les Applications de L'Explosion
Thermonucleaire, Editions Berger-Levrault, Paris, (1956).
6. Pokrovskiy, G. I., "On the Use of Nuclear Explosives for
Industrial Purposes," Gor-nyi Zhurnal, Vol. 1, pp. 29-32,
(1956); also translation AEC-tr-4005.
7. Marder, Murray, "Reds Attack Peaceful U.S. Atom Blasts,"
Washington Post, (September 4, 1958), p. 1.
8. Rawson, D., C. Boardman, and N. Jaffe-Chazan, The Environment
Created by a Nuclear Explosion in Salt, PNE-107F, (1965); D.
Rawson, Review and Summary of Some Project Gnome Results, AGU
Vol. 44, (1963), pp. 129-35; M. Nathans, Isotope Program -
Project Gnome, PNE-102F, (January 1965).
9. Werth, G., Ed., The Handcar Nuclear Explosion in Dolomite,
Lawrence Livermore National Laboratory, Livermore, CA,
UCRL-50951, (October 13,1970).
10. Crowley, B., and H. D. Glenn, The Marvel Experiment,
UCRL-72756, (October 19, 1970).
11. In the mid-1960s, the Johnson administration established a
policy that 50 percent of the field costs of any Plowshare
industrial experiment must be paid by an appropriate industrial
sponsor. In 1967, following the Gasbuggy experiment, this policy
was changed to 90 percent, which significantly discouraged any
further industrial interest in participating in Plowshare
experiments.
12. Holzer, F., GASBUGGY Experiment, UCRL-71624, (March 1969);
D. Rawson, et al., Postshot Geologic Investigations-Project
GASBUGGY, UCRL-71354, (September 1968); C. Smith, Jr., Project
GASBUGGY Gas Quality Analysis and Evaluation of Radiochemical
and Chemical Analytical Results, UCRL-50635, Rev. 1, (November
1969); L. Aamodt, "RULISON; Underground Engineering Explosive
and Emplacement Considerations," IAEA-PL-429/30), IAEA Peaceful
Nuclear Explosions II, (January 1971); C. Smith, Gas Analysis
Results for Project RULISON Production Testing Samples,
UCRL-51153, (November 1971); W. Woodruff, and R. Guido, "Project
RIO BLANCO Part I: Nuclear Operations and Chimney Reentry,"
IAEA-TC-1-4/4, IAEA Peaceful Nuclear Explosions IV, (January
1975); J. Toman, "Project RIO BLANCO Part II: Production Test
Data & Preliminary Analysis of Top Chimney/Cavity,"
IAEA-TC-1-4/5, ZAEA Peaceful Nuclear Explosions W, (January
1975).
13. Tewes, H., Survey of Gas Quality Results from Three Gas-Well
Stimulation Experiments by Nuclear Explosions, UCRL-52656,
(January 1979).
14. Dubasov, Yu. V., et al., "Nuclear Explosion Technologies:
Features of the Conduct of Nuclear Explosions for Peaceful
Purposes," Bulletin of the Center for Public Information on
Atomic Energy, (January 1994), pp. 30-35, Moscow.
15. Nuclear Explosions in the U.S.S.R. - Publication 4 -
Peaceful Uses of Nuclear Explosions, Ed. V. N. Mikhailov, p. 4,
VNIPIpromtekhnologiy and Khlopina Radium Institute, Moscow,
(1994).
16. Personal communication, Roland Timerbaev, formerly in the
Soviet Foreign Ministry.
17. Personal communication with Boris V. Litvinov, Chief Weapons
Designer at the Chelyabinsk Nuclear Weapons Laboratory, (May
1994).
18. Mikhailov, Nuclear Explosions in the U.S.S.R., p. 4 (see
endnote 15 for complete reference).
19. Holzer, A., and G. Werth, Summary of the Technical Aspects
of the U.S.-U.S.S.R. Talks of April 14-16, 1969 at Vienna,
Austria, UCID-15499, (July 1, 1969); G. Werth, Highlights of the
Second Stage of Soviet-American Technical Talks on the Use of
Peaceful Nuclear Explosions for Peaceful Purposes, UCID-15606,
(February 24, 1970); M. D. Nordyke, Technical Summary of the
Third Stage of the Soviet-American Talks on the Peaceful Uses of
Nuclear Explosions, UCRL-51113, (August 23,1971).
20. Peaceful Nuclear Explosions, Phenomenology and Status
Report, 1970, proceeding of a panel held at IAEA, (March
2-6,1970); Peaceful Nuclear Explosions II, Their Practical
Applications, proceeding of a panel held at IAEA, (January
18-22,1971); Peaceful Nuclear Explosions III, Applications,
Characteristics and Effects, proceeding of a panel held at IAEA,
(November 27-December 1, 1972); Peaceful Nuclear Explosions IV,
proceeding of a panel held at IAEA, (January 20 - 24, 1975); and
Peaceful Nuclear Explosions V, proceeding of a panel held at
IAEA, (November, 22-24, 1976).
21. Nordyke, M. D., "A Review of Soviet Data on the Peaceful
Uses of Nuclear Explosions," Annals of Nuclear Energy, Vol. 2,
pp. 657-673, (1975).
22. Borg, I. Y., "Peaceful Nuclear Explosions in Soviet Gas
Condensate Fields," LLNL Energy and Technology Review, (May
1983), UCRL-52000-83-5, pp. 30-38.
23. Scheimer, J. F., and I. Y. Borg, "Deep Seismic Sounding with
Nuclear Explosives in the Soviet Union," Science, Vol. 226, No.
4676, (November 16,1984).
24. Mikhailov, op. cit., p. 4.
25. These totals do not include a seismic event at 0900 GMT on
July 19, 1982 at seismic coordinates 62.532 N and 47.813 E about
200 km NNE of the city of Kotlas with a seismic magnitude of
4.4. This event is not listed as a PNE by documents from the
Ministry of Atomic Energy, but it is included in PNE lists
compiled by Sultanov et al. under the name "Komipetroleum" (See
D. D. Sultanov, et al., Investigation of Seismic Efficiency of
Soviet Peaceful Nuclear Explosions Conducted Under Various
Geological Conditions, Russian Academy of Sciences, Institute
for Dynamics of the Geosphere, (July 28,1993.)
26. The History of Soviet Nuclear Weapons, Draft Outline VNIIEF/VNIITF,
Moscow, 19923; U.S.S.R. Nuclear Weapons Tests and Peaceful
Nuclear Explosives, 1949 Through 1990, RFCN-VNIIEF, Sarov,
15RN5-85165-062-1, (1996).
27. Dubasov, Y. V., et al., "Underground Explosions of Nuclear
Devices for Industrial Purposes on the Territory of the U.S.S.R.
in 1965-1988," Bulletin of the Center for Public Information on
Atomic Energy, Moscow, (January 1994), pp. 18-29.
28. Mikhailov, Nuclear Explosions in the U.S.S.R., p. 67 (see
endnote 15).
29. "IAEA Clears Semipalatinsk Area Conditionally for Living,"
Nucleonics Week, (January 26,1995), pp. 6-7.
30. Seaborg, G. T., Stemming the Tide, Arms Control in the
Johnson Years, Lexington Books, (1987).
31. Dubasov, op. cit, p. 25.
32. "Bulking" refers to the increase in volume of a solid when
it is broken up into small, randomly shaped pieces.
33. Dubasov, op. cit, p. 25.
34. Stefashin, Oleg, "Unknown New Test Site," Izvestiya,
(January 23, 1991), p. 2; from JPRS-TAC-91-004, p. 33.
35. Lushin, Yuri, "A Big Secret Tor Peaceful Purposes," OGONEK,
No. 2, (January 1992), pp. 4-15.
36. Kireev, V. V., "Group Excavation by Nuclear Explosions in
Alluvial Media," IAEA-TC-1-4/14 in Peaceful Nuclear Explosions
IV, IAEA panel, pp. 399-419, (1995).
37. Micklin, P. P., "Dimensions of the Caspian Sea Problem,"
Soviet Geography, Vol. XIII, No. 9, (November 1972), pp.
589-602.
38. Dubasov, op. cit., p. 25.
39. Pankov, A., "The Pechora Will Flow into the Caspian," Vodnyy
Transport, (December 4, 1969), p. 2.
40. Mikhailov, op. cit., p. 23.
41. Gorin, V. V., et al., "Semipalatinsk Test Site: A Chronology
of Underground Nuclear Explosions and Their Primary Radiation
Effects (1961-1989)," Bulletin of the Center for Public
Information on Atomic Energy, No. 9, pp. 21-32, Moscow, (1993).
42. History of Soviet Nuclear Weapons, op. cit, p. 46.
43. Mikhailov, op. cit, p. 70.
44. Ibid., p. 91.
45. Chelyukanov, V. V., et al., "On Radiation Conditions in the
Perm Oblast," Bulletin of the Center for Public Information on
Atomic Energy, No. 2, pp. 72-74, Moscow, (1993).
46. Dubasov, op. cit, p. 25.
47. Chelyukanov, op. cit.
48. Kireev, op. cit..
49. The English language version of the LTBT prohibits any
nuclear explosion that "causes radioactive debris to be present
outside the territorial limits of the State" conducting the
explosion, whereas the Russian text refers to the presence of
"radioactive fallout." Thus, the Russian text would appear to
permit nuclear explosions which resulted in radioactive gases
that crossed borders but not nuclear explosions that led to
detectable fallout beyond the border.
50. The Peaceful Nuclear Explosions Treaty provides that no
group nuclear explosion may have any single explosion greater
than 150 kt and the total yield of any group explosion cannot
exceed 1,500 kt. These yield limits would appear to be adequate
to carry out the Kama - Pechora project.
51. Philip P. Micklin, "A Preliminary Systems Analysis of
Impacts of Proposed Soviet River Diversions On Arctic Sea Ice,"
EOS, Vol. 62, No. 19, (May 12, 1981).
52. Mikhailov, op. cit., p. 71.
53. There have been reports that the experiment was a failure
and that the dome subsided to its original level. See "'Focus'
Ne Ydalsya," in Atom bez Gripha "Sekretno": Tochki Zreniya, A.
Emel'yanenkov and V. Popov, Ed. H&P Druck, Berlin, (1992).
54. Myasnikov, K. V., et al., "Underground Explosion in the
Arctic for Peaceful Purposes," in Nuclear Explosions in the
U.S.S.R. - Publication 1: The Northern Test Site, Ed. V. N.
Mikhailov, VNIPIpromtekhnologiy and Khlopina Radium Institute,
Moscow, (1992). (See JPRS-UEQ-93-009-L).
55. Mikhailov, op. cit., p. 71.
56. Dokuchayev, M., "The Blast at Medeo," Nauka i Zhizn', No. 3,
(1967), pp. 100-8; Ya. A. Yulish, "Baipazinskiy Hydroelectric
Power Installation on the Vakhsh River," Gidrotekhnika i
Melioratsiya, No. 7, (1971), pp. 1-10.
57. Mikhailov, op. cit, p. 71.
58. Dubasov, op. cit., p. 24.
59. Orudjev, S. A., "Underground Nuclear Explosions to Stimulate
Oil Field Development," Proceeding of the 8th World Petroleum
Congress, Moscow, (June 1971).
60. Nordyke, op. cit, p. 666.
61. Mikhailov, op. cit, p. 40.
62. Ibid., pp. 40-42.
63. Ibid., p. 101.
64. For comparison purposes, the initial tritium levels in gas
from the three U.S. gas stimulation experiments were 0.7, 0.175,
and 0.028 uCi/liter in Gasbuggy, Rulison, and Rio Blanco. The
internal dose from the use of natural gas for cooking in an
unvented kitchen which had a tritium level of 0.01 uCi/liter is
estimated to be 1.3 mrem/year, or less than one percent of
natural background levels of exposure. (See Barton, et al,, "Calculatiohal
Techniques for Estimating Population Doses from Radioactivity in
Natural Gas from Nuclearly-Stimulated Wells," IAEA-TC-1-4/3 in
Peaceful Nuclear Explosions IV, IAEA Panel, (1995), pp. 343-354.
65. Nordyke, op. cit, pp. 665-666.
66. Orudjev.op. cit.
67. Ibid.
68. Yanshin, Acad. A., "The Nuclear 'Genie' is Escaping from the
Earth," Delo (Moscow), No. 13 (47), (March 1994), p. 4. Yanshin
is also Chairman of the Scientific Council of the Russian
Academy of Sciences for Problems of the Biosphere.
69. Golybov, B., "Point of View of the Experts," Atom bez Gripha
'Sekretno': Tochki Zreniya, A. Emel'yanenkov and V. Popov, Ed.
H&P Druck, Berlin, (1992), pp. 67-8.
70. Yakimets, V., "A Hundred Test Sites in the Former U.S.S.R.,"
Spasenie No. 19-20, (June 1992), p. 4.
71. Yanshin, op. cit.
72. Mikhailov, op. cit, p. 44.
73. Vasil'yev, V. G., Gas Deposits of the U.S.S.R., Moscow,
(1968), pp. 92-3.
74. Mikhailov, op. cit, p. 145.
75. Kiselev, A. Ye., and V. V. Minner, "Lithologic Composition
and Reservoir Properties of the Osin Horizon of the
Sredne-Botuobinsk Field," Nauka, (1979), pp. 76-82.
76. Kedrovskiy, O. L., On the Exploitation of Oil and Gas
Deposits in Low Permeability Reservoirs, Geologiya Nefti i Gaza,
No. 11, (1980), pp. 43-46.
77. Mikhailov, op. cit., pp. 42-46.
78. Musinov, V. I., "Production of Oil and Gas with the Aid of
Nuclear Explosions," Priroda, (1991), No. 1, pp. 25-33.
79. Kedrovskiy, O. L., M. C. Lykin, V. I. Musinov, Ye. M.
Simian, "A Study of the Influence of the Electrical Field on the
Filtration of Oil in a Low Permeability Layer," Nefty-anoe
Khozyaistvo, (1986), No. 12, pp. 45-48.
80. Dubasov, op. cit, pp. 21-22.
81. Mikhailov, op. cit., p. 42.
82. Ibid., p. 41.
83. Mikhailov, op. cit., p. 42.
84. Sultanov, D. D. et al., Investigation of Seismic Efficiency
of Soviet Peaceful Nuclear Explosion Conducted in Various
Geological Conditions, Part 1, Russian Federation Institute for
Dynamics of Geospheres, Moscow, (1993).
85. Adushkin, V. V., et al. Characteristics of Seismic Waves
from Soviet Peaceful Nuclear Explosions in Salt, UCRL-CR-120929,
(April 1995).
86. Adushkin, V. V., et al., "Overview of the Experimental Data
and Theoretical Simulations of Underground Nuclear Explosions
Decoupled by Large Air-Filled Cavities," Reports of the Russian
Academy of Sciences, Vol. 327, No. 1, (1992).
87. Vasil'ev, V. G., Gas Deposits in the Soviet Union, Nedra,
Moscow, (1968), pp. 626628.
88. Igrevskiy, V. I. and K. I. Mangyushev, Prevention and
Elimination of Oil and Gas Fountains, Nedra, Moscow, (1974).
89. Early references such as endnote 71 show the runaway well as
No. 11 in the Urt-abulak field, but later sources give the name
as IP in the Pioneer field.
90. Personal communication with B. Litvinov, (May 1994).
91. Ibid.
92. Mikhailov, op. cit., pp. 50-51.
93. Ibid. p. 151.
94. Although all sources state that Project "Magistral" was the
first nuclear explosion directed at the development of
underground storage technology, all MinAtom lists carry an event
code-named "Tavda" on October 10, 1967 as the first storage
explosion. This explosion had a yield of only 0.3 kt and was at
a depth of 172 m. It was located on the east side of the Urals,
about 70 km north-northeast of Tyumen near the Tavda River.
95. Mikhailov, op. cit, pp. 35-39. This reference gives the gas
pressure in the cavity as 8.4 Pa (Pascals). This would appear to
be an error. Since lithostatic pressure would be expected to be
about 140 atmospheres (14 megaPascals), this author has assumed
the units should have been MPa (megaPascals).
96. A 1972 paper presented at the IAEA by K. V. Myasnikov
describes testing an explosion cavity at lithostatic pressure,
presumably "Magistral," with both oil and gas, leading to the
conclusion that its effective volume for storage of liquids was
10 percent larger than its geometric volume; for gases at
lithostatic pressure it was 24 percent larger.
97. Endnote 15 says the site was in industrial exploitation for
11 years on p. 37 and 18 years in Appendix 1. Endnote 12, p. 8,
says the site was in exploitation for 18 years.
98. Although the endnote 15 text refers to these radiation
levels as "above background," they would appear to be at or near
normal levels.
99. Borg, op. cit. 100.Mikhailov, op. cit. 101.Borg, op. cit.
102.The first three explosions at "Vega" on October 16, 1982 all
had seismic magnitudes of 5.2. The last explosion was measured
at 5.4. In addition, in endnote 83, which gives the exact times
for all Vega explosions, the last one at 6:15 a.m. GMT is given
a yield of 13.5 kt. For these reasons, I have associated the
13.5 kt explosion with the last of the four in table 3.
103.Krikokhatskiy, A. S., et al., "On the Results of Nuclear
Explosions Carried Out in the Astrakhan Gas Condensate Deposit
for the Creation of Underground Storage," Bulletin of the Center
for Public Information on Atomic Energy, Moscow, (May 6, 1994),
pp. 51-53.
104.Yanshin, op. cit.
105. Mikhailov, op. cit, p. 148. An equally authoritative source
(endnote 66) states that there are three isolated areas with
dose rates of 100-200 uR/h.
IQG.Platt's Oilgram News, 60 (214), (November 5, 1982).
107.Kiryukhin, L. G., "Characteristics of the Formation of Zones
of Regional Highs in the Sub-Salt Complex of the Pre-Caspian
Depression," Petroleum Geology, 19 (4), (1981), pp. 182-186.
108.Dubasov, op. cit.
109.Benz, H. M., et al., "Deep Seismic Sounding in Northern
Eurasia," EOS, Vol. 73, No. 28, (July 14,1992), pp. 297-300.
110.Scheimer, J. F. and I. Y. Borg, "Deep Seismic Sounding with
Nuclear Explosives in the Soviet Union," Science, Vol. 226, No.
4676, (November 16,1984), pp. 787-792.
111. Endnote 15 states that two DSS profiles used PNEs fired for
other purposes, but does not identify them. Endnote 107 lists
the Oka (Neva) oil stimulation explosion on November 5,1976 as a
source for the Bortuoba-Tungus-Khaya Line.
112.Egorkin, A. V., "Studies of Mantle Structure of U.S.S.R.
Territory on Long-Range Seismic Profiles," Phys. Earth Planet
Int., Vol. 25, p. 12, (1981).
113.Egorkin, A. V. and V. V. Run, Phys. Earth Planet Int., Vol.
14, (1978), p. 262.
114.Vbrvovskiy, I. S., Seismic Studies of the Earth's Crust in
the U.S.S.R., Nedra, Moscow, (1973).
115.Zverev, S. M., and I. P. Kosminskaya, Eds., Seismic Models
of the Main Geostruc-tures of the U.S.S.R. Territory, Nauka,
Moscow, (1980).
116. Egorkin, A. V., et al., "Results of Lithospheric Studies
from Long-Range Profiles in Siberia, Seismic Studies of the
Continental Lithosphere," Tectonophysics, 140, (1987), pp.
29-47.
117.Ryaboy, V., Upper Mantle Structure Studies by Explosion
Seismology in the U.S.S.R., (Delphic Press, 1989).
118. Benz, op. cit.
119. Personal Communication, V. Simonenko, (1993). 120.Dubasov,
op. cit.
121.Bychenkov, V. A, "Effect of the Slit Position and Width on
the Amount of Rock Crushed by an Explosion,"
Fiziko-Tekhnicheskie Problemy Razrabotki Iskopaemykh, No. 2,
(1973), pp. 53-58.
122.Imenitov, V. R., "Questions on the Use of Nuclear Explosions
for Underground Ore Production," Gornyi Zhurnal, No. 12, (1973),
pp. 33-36.
123.Nordyke, op. cit., (see endnote 19).
124.Mikhailov, op. cit., p. 64.
125.1bid., p. 91.
126.1bid., p. 64.
127.Ibid., pp. 63-66.
128."Leakage of Radiation after 1974 Explosion on Kola
Peninsula," U.S.S.R. Today, (October 28,1991), p. 20.
129.Vasirev, A. P., N. K. Prikhod'ko, and V. A. Simonenko,
"Underground Nuclear Explosions for Improvement of Ecological
Conditions," Priroda, (1991), No. 2, pp. 36 - 42.
130. Mikhailov, op. cit, pp. 54-57.
131. Wheeler, J. A., "Plutonium Breeding, Collection of
Materials," in Industrial Uses of Nuclear Explosives, UCRL-5253,
(September 8, 1958), pp. 79-81.
132.Nathans, M. W., "Recovery of Isotopes," in Proceedings of
the Second Plowshare Symposium, Part III, UCRL-5677, (May, 14,
1959), pp. 24-32.
133. Violet, C. E., "Project Gnome," in Proceedings of the
Second Plowshare Symposium, Part III, UCRL-5677, (May, 14,1959),
pp. 4-12.
134. Personal communication, Vadim Simonenko. 135.Mikhailov, op.
cit, p. 146.
136.U.S.S.R. Nuclear Weapons Tests and Peaceful Nuclear
Explosions, 1949 through 1990, RFCN-VNIIEF, Sarov,
ISBM-85165-062-1, (1996).
137.Higgins, G. H. and T. R. Butkovich, Effect of Water Content,
Yield, Medium, and Depth of Burst of Cavity Radii, Lawrence
Livermore Laboratory, UCRL- 50203, (February 1967).
138.Krivokhatskiy, Yu. V., et al., "Radiation Manifestations of
Underground Explosions for Peaceful Purposes at the Bolshoy
Azgir Deposit," Bulletin of the Center for Public Information on
Atomic Energy, 9/93, Moscow, (1994), pp. 49-59.
139.1bid.
140. In this context, it was argued that the radius of the
cavity in meters would have to be equal to 30 - 40 times the
cube root of the yield of the explosion being decoupled in order
to fully decouple.
141.Werth, G. and P. Randolph, "The SALMON Seismic Experiment,"
J Geophys. Res., 71:3405-13, (July 1966); D. Rawson, et al.,
"Review of the SALMON Experiment - A Nuclear Explosion in SALT,"
Naturwissenschaften, Vol. 54: 525-31, (October 1967); and D.
Rawson, et al., "Post-Explosion Environment resulting from the
SALMON Event," J. Geophys. Res., Vol. 71:3507-21, (July 1966).
142.This decoupling factor is based on relatively close-in
seismic data (less than 110 km), which are the only data
available.
143. Endnote 86 gives the yield of this explosion as 8 kt, based
on a hydrodynamic measurement in the cavity. However, a chief
weapons designer at Arzamas Laboratory has privately stated (see
endnote 144) that the yield, measured by a much more reliable
method (radiochemistry) was 11.5 kt. The yield given in the text
and used in this paper is the one provided by MinAtom in endnote
15.
144. Glenn, L. A. and P. Goldstein, "Seismic Decoupling with
Chemical and Nuclear Explosions in Salt," J. Geo. Res., Vol. 99,
(June 10,1994), pp. 11723-30.
145.Personal communication, Vitaly Adushkin, (September 12,
1996).
146.Mikhailov, op. cit., pp. 58-60.
147."Secret Nuclear Test in Donbass Mine Revealed," Kiev Radio
Ukraine World Service, (August 5, 1992), from JPRS-TAC-92-026,
(August 31, 1992), pp. 41-42.
148.Goncharov, 'Viktor and Sergei Peteshov, "The Experiment
under Code-Name "Cleavage:," in Atom bez Gripha "Sekretno:
Tochki Zreniya, pp. 65-67, Moscow-Berlin, (1992).
U9.Izvestiya, (June 28, 1992).
150.Paper CCD/388 (August 24, 1972) in Documents on Disarmament
1972, pp. 590615.
151.Progress and Problems in Seismic Verification Research,
Defense Advanced Research Projects Agency, (TIO-73-3), (1973),
p. 79.
l52.Nezavisimaya Gazeta, (March 5,1992), p. 6.
153.Black, S., and B. Morel, "Rational Disposal of Chemical
Weapons," Nature, No. 360, (December 17, 1992), pp 621-622.
154.Klimenko, V., "A New Look at the Problems of Weapons of Mass
Destruction in Russia and the Newly Independent States," in
Nuclear Control, No. 4, (April 1995), p. 21.
155.Teletype message from H. Brown, LRL, to Starbird, AEC, Ideas
for PNE Devices under a Moratorium, COPD 58-73, (September
25,1958).
156.Teletype message from Starbird, AEC, to E. Teller, LRL,
L-2347-58, (November 7, 1958).
157.Conference on the Discontinuance of Nuclear Weapons Tests,
GEN/DNT PV.25, (December 15,1958), p. 11.
158. Conference on the Discontinuance of Nuclear Weapons Tests,
GEN/DNT PV.26, (December 16,1958), p. 23.
159.Jacobson and Stein, p. 156, and Pravda, (December 26,1958),
pp. 9-10.
160."Control and Reduction," Testimony of Edward Teller in
Hearings before a Subcommittee of the Senate Committee of
Foreign Relations, (March 16, 1958).
161.Conference on the Discontinuance of Nuclear Weapons Tests,
GEN/DNT PV.46, (January 30,1958), p. 7.
162.1bid., pp. 8-10.
163.Conference on the Discontinuance of Nuclear Weapons Tests,
GEN/DNT PV.60, (February 23, 1959), p. 30.
164.1bid., pp. 33-34.
165.Statement by the United States Representative, Henry Cabot
Lodge, to the First Committee of the General Assembly, (October
14,1959), in DOD, 1945-59, p. 1,493.
166. Conference on the Discontinuance of Nuclear Weapons Tests,
GEN/DNT PV.274, (March 21, 1961), pp. 16-27.
167.Seaborg, Glenn T, Kennedy, Khrushchev and the Test Ban,
Univ. of Calif. Press, (1981), p. 244
168.1bid., p. 268.
169.Nordyke, M. D., Technical Summary of the Third Stage of the
Soviet-American Talks on the Peaceful Uses of Nuclear
Explosions, UCRL-51113, (August 23,1971).
170.A plan for nuclear excavation of a canal connecting the Gulf
of Siam with the Strait of Malacca in Southern Thailand was
jointly studied by the U.S. and Thai Governments in 1973-75 (see
IAEA reference below, January 1995). Similarly, a plan for using
nuclear excavation to connect the Qattara Depression and the
Mediterranean Sea was jointly studied by the U.S., West Germany,
and Egypt in the mid-1970s ("Development of the Qattara Project,
Egypt," in Peaceful Nuclear Explosions V, IAEA-TC-81-5/6,
November 22-24, 1976).
171. See Peaceful Nuclear Explosions, Phenomenology and Status
Report, (1970), (March 2-6, 1970); Peaceful Nuclear Explosions
II, Their Practical Application, 19-22 (January 1971); Peaceful
Nuclear Explosions III, Applications, Characteristics and
Effects, (November 27-December 1, 1972); Peaceful Nuclear
Explosions TV, (January 20-24,1975); Peaceful Nuclear Explosions
V, (November 22-24,1976).
172.1bid.
173.Smith, R., "Possible Nuclear Arms Test by India Concerns ,"
Washington Post, (December 16,1995), p. A17.
174. Gupta, V. and F. Pabian, "Investigating the Allegations of
Indian Nuclear Test Preparations in the Rajasthan Desert,"
Science Global Security, Vol. 6(2), (1997), pp. 101-188.
175.Hoff, R. W. and E. K. Hulet, "The Recovery and Study of
Heavy Nuclides in a Nuclear Explosion - The HUTCH Event," in
Engineering with Nuclear Explosives, Proc. ANS Symp., Las Vegas,
(January 1970).
176.Mikhailov, op. cit, p. 44.
APPENDIX A
PEACEFUL NUCLEAR EXPLOSIONS IN THE SOVIET UNION
(BY DATE)
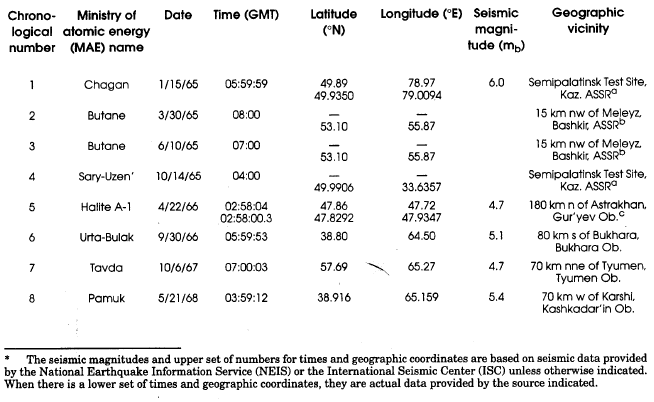
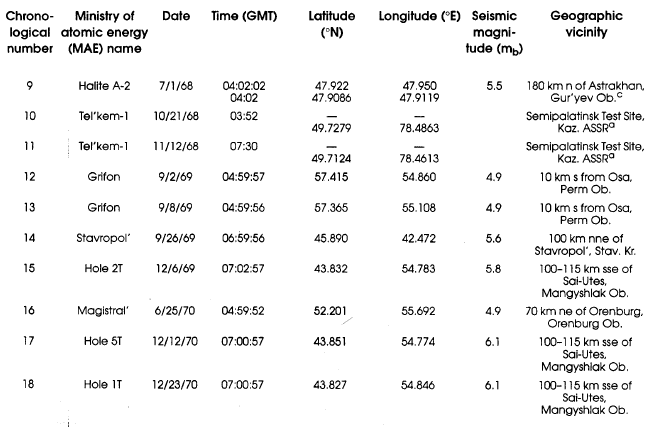
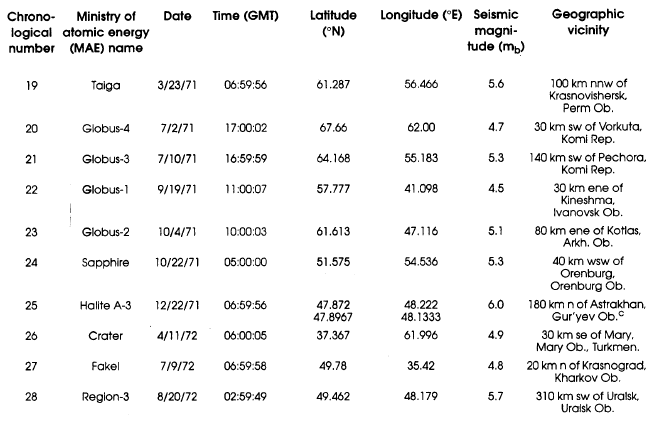
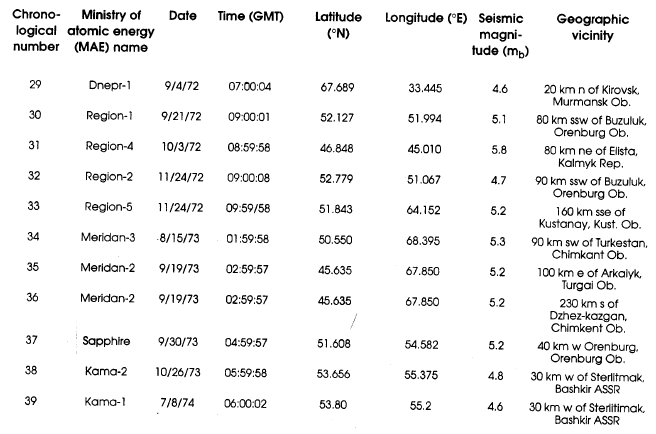
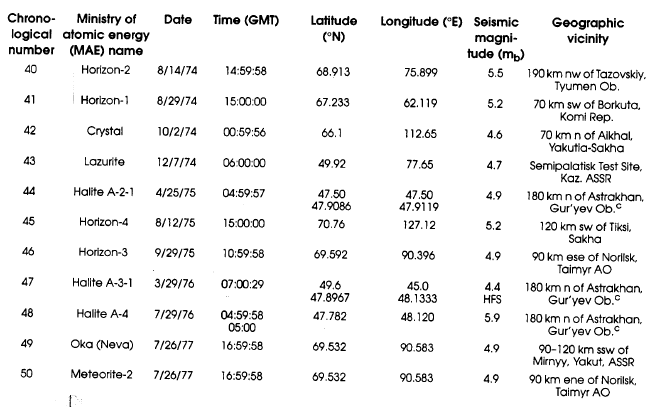
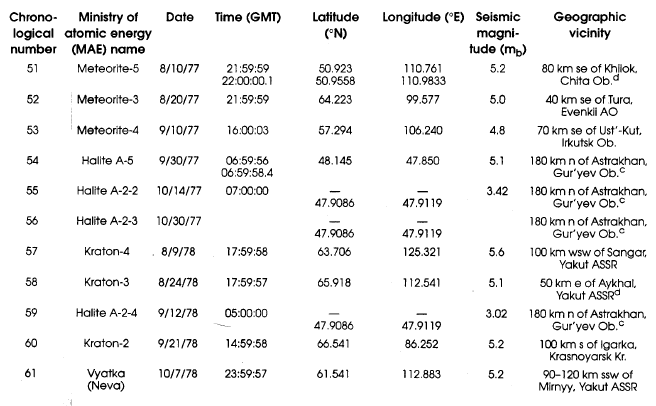
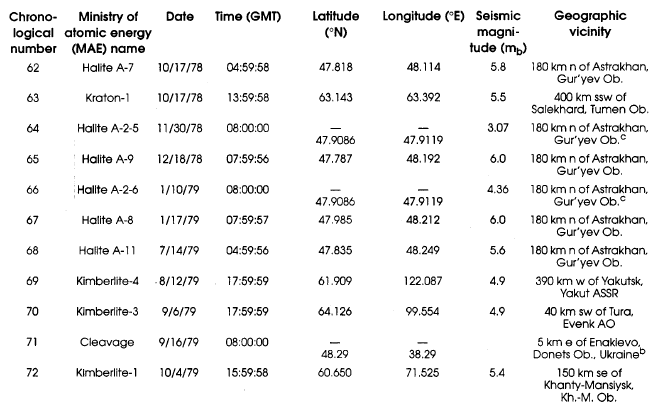
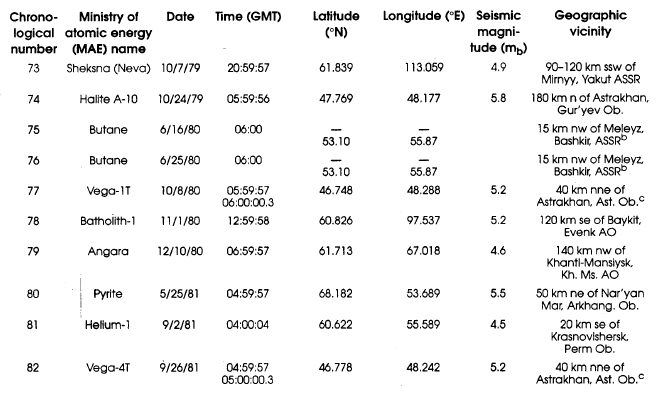
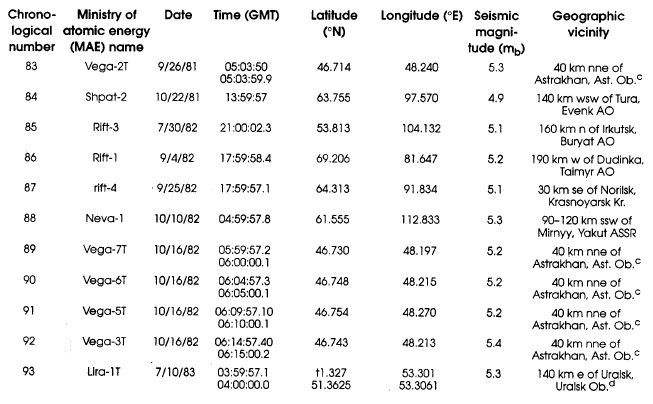
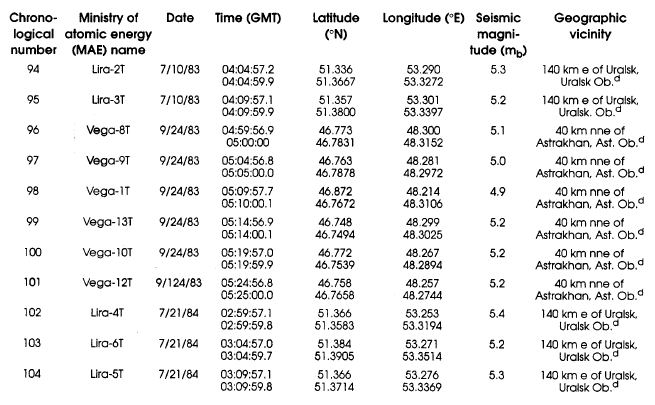
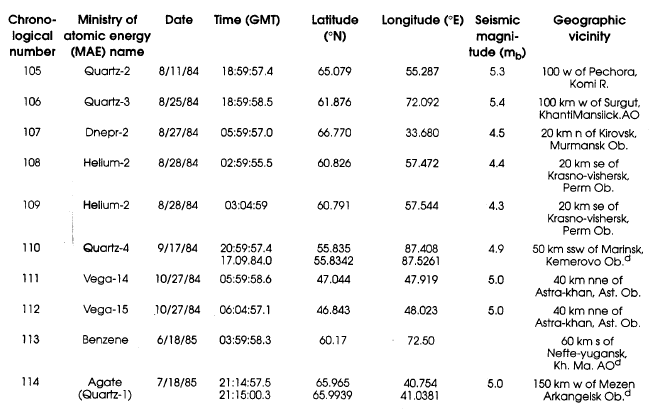
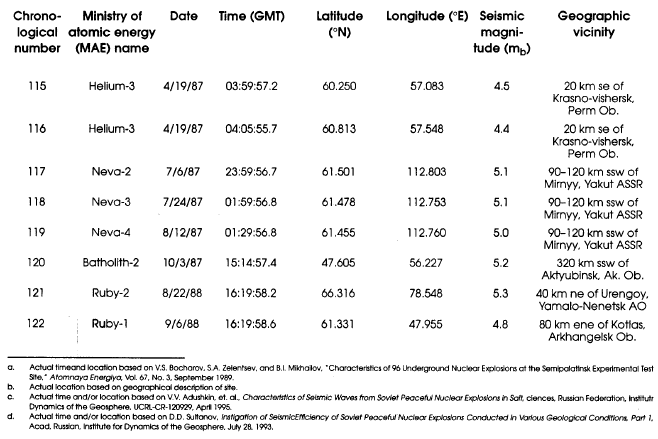
APPENDIX B
PEACEFUL NUCLEAR EXPLOSIONS IN THE SOVIET UNION
(BY PURPOSE)
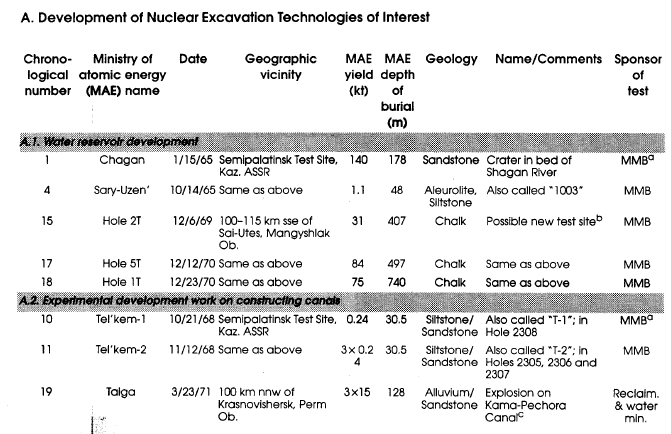
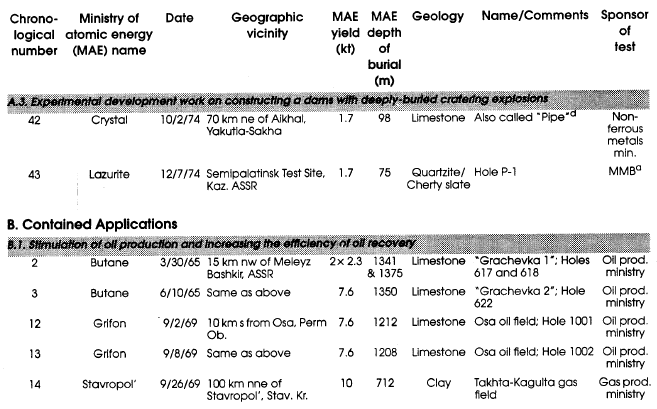
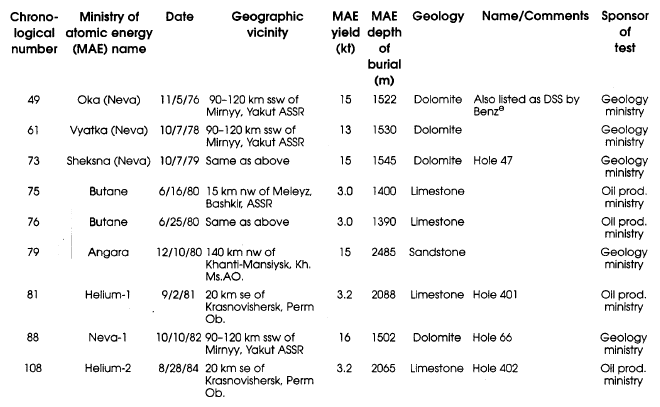
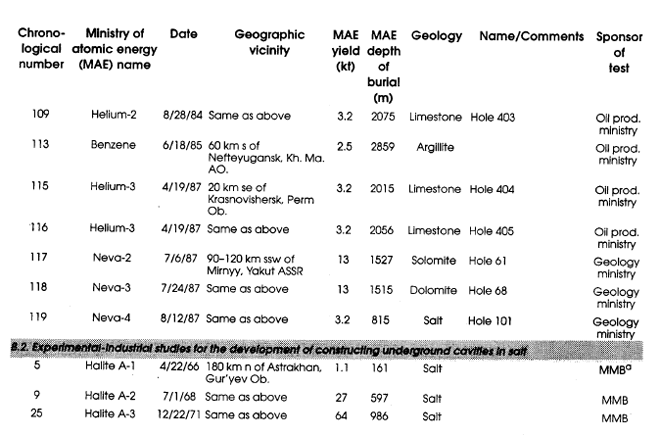
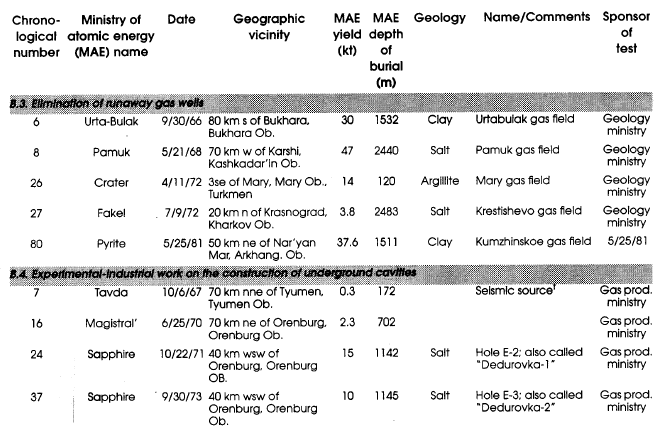
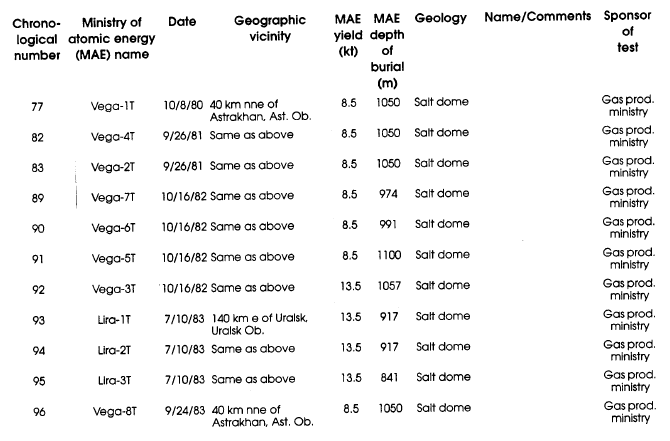
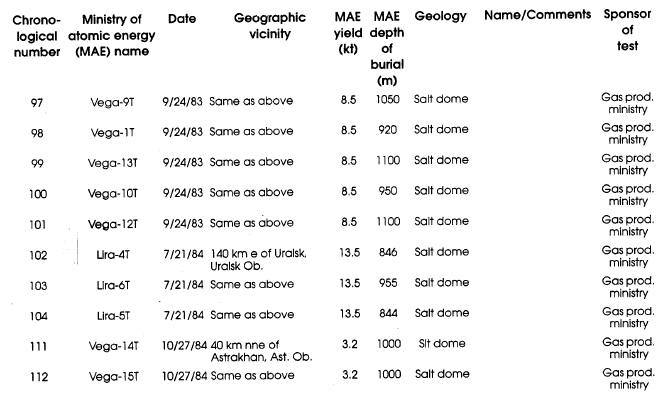
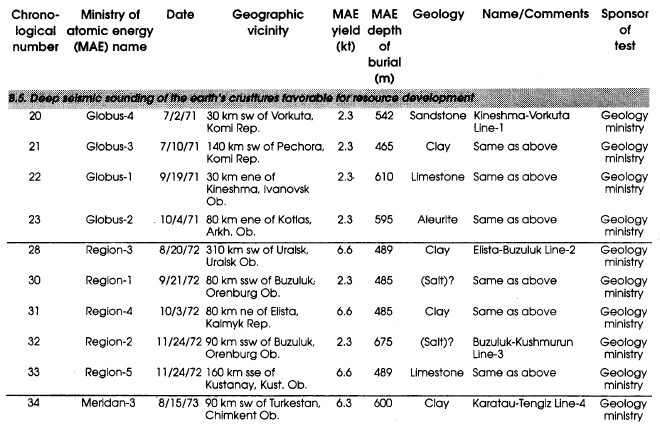
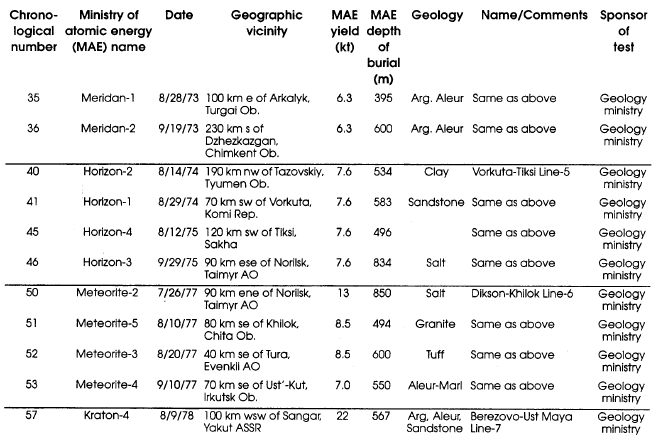
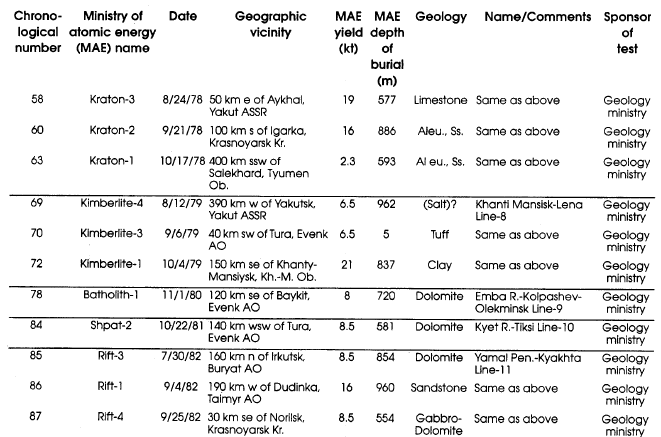
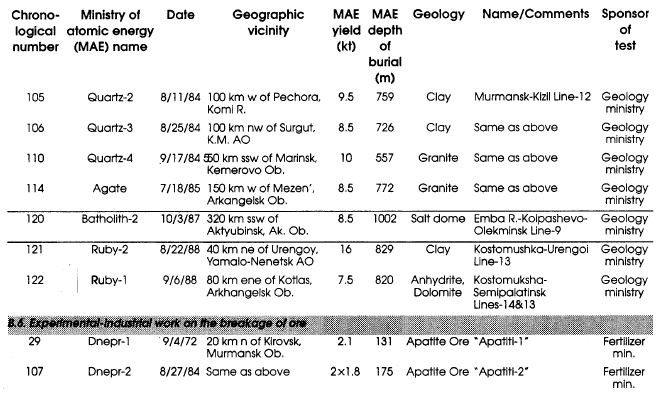
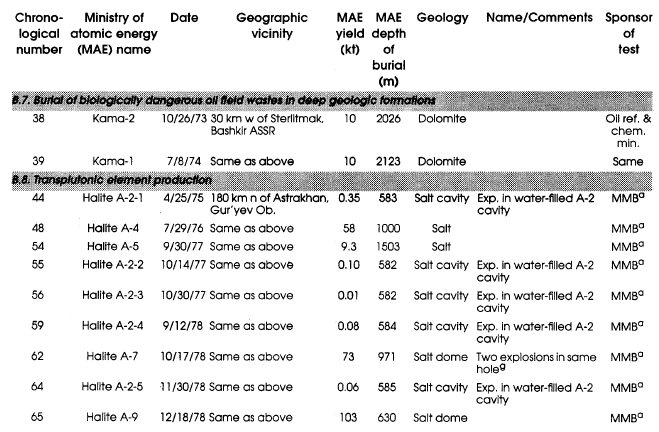
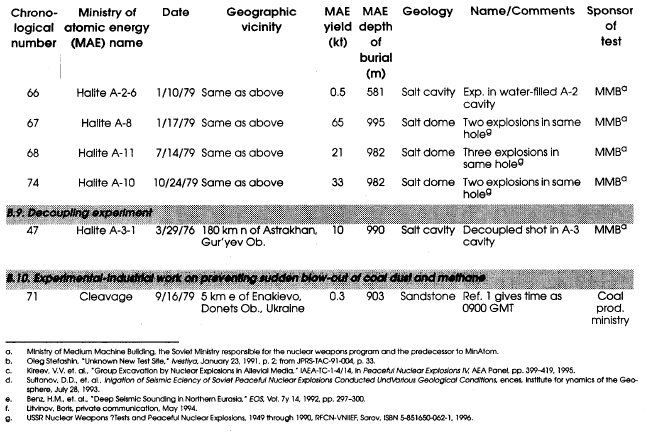
APPENDIX C
THE SOVIET PROGRAM TO DEVELOP NUCLEAR EXPLOSIVES
FOR PEACEFUL USES
The Russian Ministry of Atomic Energy (MinAtom)
recently released a comprehensive list of all nuclear explosions
conducted by the Soviet Union.1 Included is a list of the date,
test-site area location, emplacement hole, or tunnel designation for
each explosion.
In addition, for each explosion, the list includes
the general purpose and yield or yield range of the test. If the
explosion involved multiple devices fired simultaneously, the
purpose and yield range of each device test is given.
This list
reveals that during the life of the Program for the Use of Nuclear
Explosions in the National Economy, the Soviet Union carried out 40
device-development tests at its nuclear weapons test sites to
develop special nuclear explosives or emplacement techniques for
such applications. Table C.l is a list of the PNE device-development
explosions carried out for the development of such explosives.
All but two of these PNE device-development tests were at the
Degelen, Sary-Uzen, or Balapan areas of the Semipalatinsk Test Site
(STS).
The other two were at the Novaya Zemlya Test Site. As noted
in table C.1, three of these explosions, on 5/28/67, 10/17/67, and
9/11/69, consisted of two PNE device-development tests fired
simultaneously in the same tunnel complex. Another five PNE
device-development tests were fired simultaneously along with one or
more nuclear weapons tests.
Also shown in table C.1 is the seismic body wave magnitude for 37 of
these explosions as reported by the International Seismic Center (ISC).
Three were presumably of sufficiently low yield that they were not
detected and identified as events by the ISC.
Supplemental data on the yield and/or depth of burial (DoB) of some
of these events were reported earlier by two other Russian
sources,2-3 both associated with MinAtom. Endnote 2 provided a list
of the date, location, and geology of some 96 underground explosions
at STS from 1961 through 1972. Exact yields were provided for 22,
with only a yield range for the remainder.
Yields given for the same
events as in endnote 1 were, with one exception, the same.4 Endnote
3 provided a comprehensive list of all the underground explosions
fired at STS from 1961 through 1989, including the 96 that were
listed in endnote 2.
Yields and yield ranges were given for all
other events, which also agreed with endnotes 1 and 2 with a few
exceptions.5
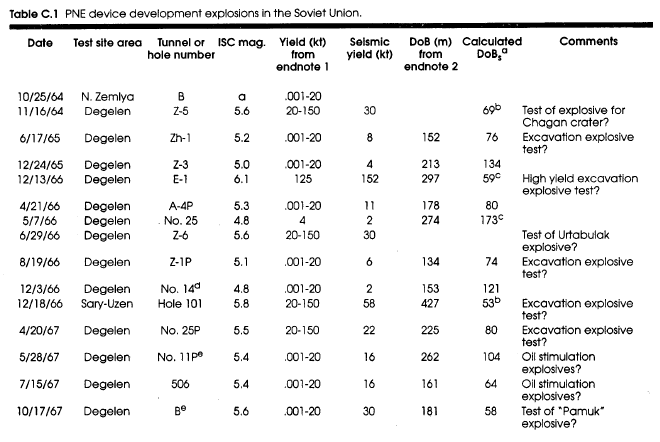
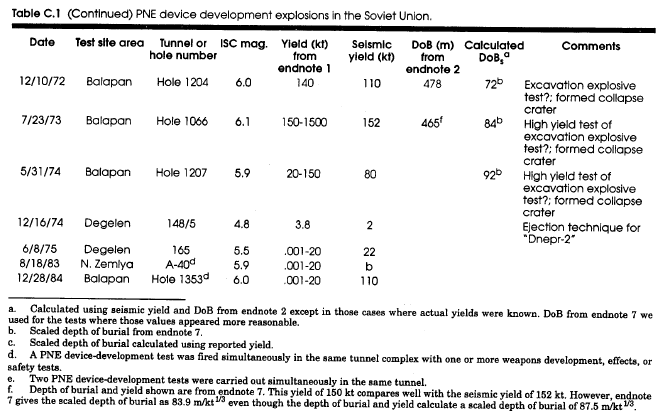
Using the yields from 19 explosions in endnote 2, all but four of
which were weapons-development or effects tests, and their ISC
seismic magnitudes, Vergino6 calculated the following general
yield-magnitude relation for explosions in any of the three areas of
the STS:

where

and

...which has a two-sigma error of about ±1.7 x.
The seismic yields
shown in the sixth column of table C.1 for the 32 PNE
device-development explosions at STS with ISC magnitudes were
calculated using this equation.
Endnote 2 provided a depth of burial for many of the PNE
device-development tests listed in table C.1, which are shown in
column seven. Using the seismic yield (or actual yields if known),
the cube-root scaled depths of burial were calculated as shown in
column eight, subject to the exceptions noted in the table, which
arose from a recent publication by Adushkin and Spivak of the
Russian Institute for Dynamics of the Geosphere.7
This report provides data on a large
number of Russian nuclear explosions that were buried at depths that
resulted in cratering or other disruption of the earth's surface. In
addition to those PNE cratering explosions discussed in Section III
of the main report, Adushkin and Spivak also include data on many
such explosions at the STS, including seven of the PNE
device-development tests listed in table C.1.
In some cases, their
numbers are consistent with those provided by endnotes 1-3, but in
others, they are significantly different.
Of particular note are the following
data:
-
For the 12-18-66 Hole 101 event
in the Sary-Uzen Area of STS, Adushkin and Spivak give the
yield as -80 kt, which is consistent with the seismic yield,
but they give the depth of burial as 228 m, significantly
smaller than the 427 m in endnote 2. They also describe the
geology as sandstone overlain by a clay layer 40 m thick and
sandy loam 7 m thick, rather than pro-phyrite as given in
endnote 2. Adushkin and Spivak's numbers indicate a scaled
depth of burial of only 59 m/kt1/3, which is
consistent with the cratering action and venting of the
explosion described by them. Endnote 3 also indicates that
this explosion dynamically vented. The crater produced by
the explosion had a radius of 145 m and a depth of about 15
m, but with a 10-m-high mound in the center.
-
For the 11-04-70 Hole 125 event
also in the Sary-Uzen Area of STS, Adushkin and Spivak give
the yield as 19 kt, which is consistent with the seismic
yield, but they give the depth of burial as 151.3 m,
significantly smaller than the 249 m in endnote 2. They
describe the geology as a por-phyritic massif overlain by
sandy gravel and loam deposits 10-27 m thick, which is
consistent with endnote 2. Adushkin and Spivak's numbers
indicate a scaled depth of burial of only 57 m/kt1/3, which
is consistent with the cratering action and venting of the
explosion described by them. Endnote 3 also indicates that
this explosion vented. The crater produced by the explosion
had a radius of 95-105 m and a depth of about 17.5 m, but
with an 8-m-high mound in the center. As indicated in the
main report, this explosion was the final test of the
explosives used for the Taiga row-cratering explosion on the
alignment of the Pechora-Kama Canal.
-
For the 12-10-72 Hole 1204 event
in the Balapan Area of STS, Adushkin and Spivak give the
yield as "about 150 kt," which is consistent with the yield
of 140 kt given by endnote 1, but they give the depth of
burial as 378 m, significantly smaller than the 478 m in
endnote 2. The geology is described as a sand-tuff
formation, overlain by 20 m of alluvium. The explosion
produced a dome that rose to 32 m and then collapsed,
resulting in an early dynamic vent of radioactive gases and
a collapse crater with a radius of 72 m and a depth of 26 m.
-
For the 07-23-73 Hole 1066 event
in the Balapan Area of STS, Adushkin and Spivak give the
yield as 150 kt and the depth of burial as 465 m. The
explosion was fired in a granite massif overlain by alluvial
clay and sandy loam 13 m thick. The explosion in the hard
rock produced a dome that rose to a height of 19 m before
collapsing, but there was no prompt vent. It resulted in a
collapse crater with a radius of 110 m and a depth of 14 m.
-
For the 05-31-74 Hole 1207 event
in the Balapan Area of STS, Adushkin and Spivak give only a
scaled depth of burial of 92 m/kt1/3 and indicate that the
explosion was fired in a schist-type rock medium. Endnote 3
reports that the explosion was completely contained, as was
Hole 1066, but it also produced a collapse crater with a
radius of 98 m and a depth of 4.5 m.
It is of significance to note that many
of the PNE device-development tests in table C.1 were carried out at
scaled depths of burial much less than that required for complete
containment of the dynamic effects of the explosion and to prevent
prompt venting of the radioactive gases from the nuclear explosion.
Although the U.S. has essentially no
experience with nuclear explosions at scaled depths of burial
between 60 and 90 m/kt1/3, it is expected that nuclear explosions in
this range would result in the creation of a dome, prompt venting of
radioactive gases, and a major disruption of the earth's surface,
much as Adushkin and Spivak have described.
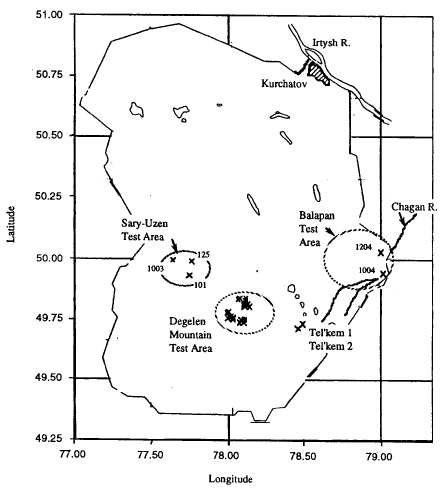
Figure C.1:
Map of the
Semipalatinsk Test Site (STS) showing the three major areas
and the location of
the PNE device-development explosions as well as the cratering
explosions
carried out at STS as
part of the nuclear excavation technology development program
discussed in the main report.
The use of such shallow scaled depths of burial with their high
probability of releasing all the radioactive gases from the nuclear
explosion would suggest that these explosions may well have been
associated with the development of new families of low-fission
excavation explosives.
As discussed in the main report, the Soviet PNE Program developed
special nuclear explosives or emplacement techniques for four
general purposes: low-fission explosives for excavation projects;
small-diameter, high pressure and temperature explosives for closure
of gas well fires; small-diameter, low-tritium explosives for
hydrocarbon stimulation applications; and techniques for ejection of
fission products far from the explosion site.
With these comments and the schedule of PNEs projects discussed in
the main report in mind, the last column in table C.1 presents
comments on some of these PNE device-development tests and
speculation on their possible purposes.
Figure C.1 is a map of the Semipalatinsk Test Site showing the
location of the three major testing areas - Degelen Mountains,
Balapan, and Sary-Uzen - as well as the locations as given in
endnote 3 of most of the PNE device-development tests listed in
table C.1.
Also shown in figure C.1 are the locations of the four
nuclear cratering explosions at STS that were described in the main
report: the "1004" Chagan crater, the "1003" Sary-Uzen crater,
TelTcems 1, and Tel'kems 2.
NOTES AND REFERENCES
1. USSR Nuclear Weapons Tests and
Peaceful Nuclear Explosions - 1949 through 1990, RFCN-VNIIEF,
Sarov, 1996; ISBN 5-85165-062-1.
2. Bocharov, V. S., S. A. Zelentsev, and B. I. Mikhailov,
"Characteristics of 96 Underground Nuclear Explosions at the
Semipalatinsk Experimental Test Site," Atomnaya Energiya, Vol.
67, No. 3, (1989), pp. 210-214.
3. Gorin, V. V., et. al., "The Semipalatinsk Test Site:
Chronology of Underground Nuclear Explosions and Their Primary
Radiation Effects," Bulletin of the Center for Public
Information on Atomic Energy, No. 9, (1993), pp. 21-32.
4. Endnote 1 gave the yield of the PNE test on 3/28/72 as being
in the range of 0.00120 kt whereas endnotes 2 and 3 give it as 6
kt. It may be significant that the PNE test was fired
simultaneously with two weapons-related tests, and the 6 kt is
the total yield.
5. Endnote 3 only gives a yield range of <20 kt for the PNE
event of 12/16/74 whereas endnote 1 gives the yield as 3.8 kt.
6. Vergino, E. S., "Soviet Test Yields," EOS, Vol. 70, No. 48,
(November 28,1989).
7. Adushkin, V. V. and A. A. Spivak, Geologic Characterization
and Mechanics of Underground Nuclear Explosions, Defense Nuclear
Agency Contract No. DNA 001-930026, (June 1994).
|