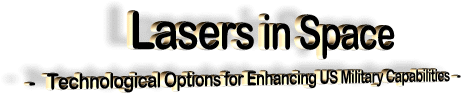
by Mark E. Rogers, Lieutenant Colonel, USAF
November 1997
Occasional Paper No. 2
Center for Strategy and Technology
Air War College
Maxwell Air Force Base, Alabama
from
AirUniversityCenterForStrategyAndTechnology
Website
The Occasional Papers series was established by the Center for
Strategy and Technology as a forum for research on topics that
reflect long-term strategic thinking about technology and its
implications for U.S. national security. Copies of No. 2 in this
series are available from the Center for Strategy and Technology,
Air War College, 325 Chennault Circle, Maxwell AFB, Montgomery,
Alabama 36112. The fax number is (334) 953-1988; phone (334)
953-2384.
Occasional Paper No. 2
Center for Strategy and Technology
Air War College
Air University
Maxwell Air Force Base, Alabama 36112 |
Contents
-
Why Lasers In Space?
-
Exploitable Characteristics Of
Space
-
Exploitable Characteristics Of
Lasers
-
A Taxonomy For Lasers In Space
-
Strategic Planning Studies
-
Criteria For Evaluating The
Concepts
-
Review And Scoring Of Concepts
-
Space-based Laser Target
Designators
-
Space-based Battlefield
Illumination
-
Moving Concepts Into The Field
-
Conclusions And
Recommendations
Disclaimer
The views expressed in this publication are those of the author and
do not reflect the official policy or position of the United States
Government, Department of Defense, or the Air War College Center for
Strategy and Technology.
Back to Contents
The Author
Lieutenant Colonel Mark Rogers, USAF, a 1976 graduate of the USAF
Academy where he majored in physics, has been involved in
defense-oriented research and development for the past twenty years,
focusing primarily on applications of lasers for military systems.
His background includes test range support for future space programs
and capabilities analysis for optical tracking systems while at
Vandenberg AFB. After completing his MS and Ph.D. in laser/optics,
he conducted and managed research on various high energy laser
weapons concepts at the AF Weapons Laboratory, including coupled
laser technology for space-based lasers as part of the Strategic
Defense Initiative. While teaching undergraduate physics at the USAF
Academy, he led a research team investigating stimulated Brillouin
scattering and stimulated Raman scattering for possible use in
nonlinearly coupling laser devices.
He headed a laser biophysics
team at the Armstrong Laboratory, where they established new safety
thresholds for sub-nanosecond pulses and near infrared wavelengths
as well as studied various nonlethal technologies based on optical
systems. Prior to entering the Air War College, he served as the
deputy chief scientist for Armstrong Laboratory, spearheading the
Lab’s information warfare program and overseeing multiple basic
research and scientific personnel programs. His current interests
include directed energy weapons, nonlinear dynamics, and
laser-tissue interactions. A 1997 graduate of the Air War College,
Lt. Colonel Rogers conducted this research under the auspices of the
Center. His current assignment is in the Pentagon.
Back to Contents
Abstract
The emerging importance of space-based systems is matched by the
maturing of laser technology, giving a potential synergy to enhance
military capability. For example, global awareness is one of the AF
goals to give the US military the competitive advantage in future
conflicts. Obtaining global awareness requires a tremendous amount
of information being acquired and transferred over vast distances.
Space-based laser communication satellites offer the potential of
greatly increased data rates, which is just one example of how
lasers in space could significantly improve US military
capabilities.
Recent strategic planning studies have identified various concepts
for lasers in space, including both laser weapons and collateral
applications such as communication and remote sensing. Four
functional classes of systems (enabling, information-gathering,
information-relaying, and energy delivery) serve to organize the
various concepts and relate them to the new AF core competencies as
well as the traditional AF roles.
This study analyzes these
concepts, scoring them for technical feasibility, technical
maturity, operational enhancement and operational cost. The most
promising concepts include space-based laser target designation,
space-based battlefield illumination, laser communication, and
active remote sensing for battle damage assessment and weather
characterization. Several strategies can accelerate the development
of space-based laser systems, such as using the new AF battlelabs
and advanced technology demonstrations.
Back to Contents
I. WHY LASERS IN SPACE?
Both laser technology and space operations have matured
substantially in the recent decades, offering synergistic
possibilities of using lasers from space-based platforms to improve
US military capabilities. Coherent laser light offers a number of
unique advantages as does the space environment, permitting
speed-of-light applications such as optical communication,
illumination, target designation, active remote sensing and
high-energy weapons. Many of these concepts have been discussed in
recent strategic studies, but it will take innovative leadership and
close cooperation between researchers and operators to bring the
concepts from the laboratory to the field.
One of the recent themes in US military thought has been achieving
global awareness in order to establish dominant battlespace
awareness. According to General Ronald Fogleman, AF Chief of Staff,
“The reality is that in the first quarter of the 21st century it
will become possible to find, fix or track and target anything that
moves on the surface of the earth.”1
Whomever has such awareness,
the theory goes, will have the upper hand in any military operation.
Awareness equates to the possession of adequate information.
Achieving global awareness will require obtaining, processing, and
relaying massive amounts of information in near-real time across
vast distances. Space-based laser systems bring many unique
characteristics to the battlefield, and thus represent powerful
tools in achieving global awareness.
Each of the new Air Force core competencies — Air and Space
Superiority, Global Attack, Rapid Global Mobility, Precision
Engagement, Information Superiority, and Agile Combat Support —
highlights an area of expertise for the AF in accomplishing both
warfighting and military-operations-other-than-war (MOOTW) missions.
As discussed more fully in a later section, lasers in space can
enhance each of these competencies. (A comprehensive list of
acronyms is included at the end of this report.) Space-based laser
systems offer unique opportunities to help the warfighters of the AF
and the other services achieve these missions.
The Department of Defense and NASA are testing various space-based
laser concepts, many of which have high military utility. While some
projects are good candidates for collaboration, the overall
development is ad hoc. There is a clear need for more overarching
coordination across the agencies and between the researchers and the warfighters. This study proposes a common framework for lasers in
space, and should serve as a catalyst to further cooperative
development of the more attractive concepts.
Objectives
The thesis of this study is that many emerging military requirements
can be met through the use of laser systems deployed on space
platforms. Laser technology has matured sufficiently in the past
decade to provide highly reliable, cost-effective, energy-efficient
and wavelength-flexible systems that can be applied to a variety of
missions, such as remote sensing and communication. Access to space
is maturing with new launch vehicles on the horizon. The unique
characteristics of the space environment greatly enhance the utility
of deploying lasers in space. These include the lack of any medium
to attenuate the beam and the ready access to the entire global
surface. This synergy of lasers in space offers the warfighter a new
and vastly more competitive tool in future conflicts. However,
technology developers must move aggressively to field prototypes
that demonstrate the capabilities and potential of space-based laser
systems for a variety of missions. The various avenues to expedite
bringing the most promising concepts into fielded systems is the
final focus of this report.
In an increasingly resource-constrained environment, the Air Force
must successfully blend strategy and technology. One straightforward
definition of strategy is “a broad concept, embracing an objective,
resources, and a plan for using those resources to achieve the
objective.” 2 There are two relevant types of strategies.
-
First,
acquisition strategy applies R&D resources (funds, manpower,
facilities, etc.) to develop new technologies that match operational
deficiencies identified by the warfighting commands.
-
Second,
operational strategy examines the threats to the security and
national interests of the US and its allies, matches current
capabilities against the threats to achieve military objectives, and
highlights areas where improvements in capabilities could enhance
military success.
It is at this point that the two strategies
interact. The warfighter must face any conflict with the tools at
hand, striving for victory with diligence and ingenuity. The
military researcher must work with equal diligence and ingenuity to
find new or more effective tools for achieving military objectives,
which in some cases will require new technology and in others might
mean repackaging existing technology into new systems. It is
increasingly important that the system developers and the
operational users work closely together. One new AF attempt to
generate this synergy is the concept of battle labs that are
discussed at the end of this report.
Scope
The field of laser technology has greatly expanded since the laser
was first demonstrated in 1960. Innovative minds have found many
applications of these technologies, including active remote sensing,
active imaging, optical communication, power beaming, and
high-energy weapons. Since the early 1960s, the complexity of the
military missions has dramatically increased, with more diverse
theaters of operation, expanded spectrums of conflict, and
tremendously increased requirements for information delivered in
almost immediately to the warfighter. It would be impossible in a
short report to comprehensively address all the unique aspects of
lasers in the space environment as well as the potential military
applications.
The scope of this paper is limited to surveying a
subset of “lasers in space” concepts to establish a basis on which
they can be compared and development decisions can be made. Each
concept could be examined in more depth, and some of the concepts
have been discussed in other, more focused reports, but that is
beyond the scope of this report. Also, the operational applications
could be discussed in more detail, which would lead to concepts of
operations (CONOPS) that consider operational employment, doctrinal
implications, constraints, proper force size, interfaces with other
systems, and so forth. Again, this type of discussion is beyond the
scope of this study. While it is impossible to give sufficient
detail about each concept to fully explain the range of benefits and
costs, this discussion will give the reader a firm understanding of
the relevant technological issues.
In the near term, most applications for lasers in the space
environment involve non-weapons systems. Although this study
discusses laser weapons in some detail, it focuses on non-weapons
applications that could be developed in the near future to enhance
the warfighters’ capability. As the analysis will show, a plethora
of maturing concepts exist that can increase military effectiveness.
The intended audience consists of the individuals in both the
research laboratories and the operational commands, including the
innovators in the battlelabs, who are building their program plans
and looking to the future technological and operational requirements
of the Air Force. Hopefully, these groups will find value in the
study of the integration of laser technology in the space
environment with the needs of the warfighter. However, the scientist
at the bench will find the technical details lacking and the
warfighter will find the operational details inadequate. This study
serves simply as a compilation of various concepts for space-based
laser systems and a brief analysis of the most likely near-term
concepts.
Military Uses of Space
Access to outer space began in the 1950s with the Soviet launch of
Sputnik I on 4 October 1957 and the US launch of Explorer I on 31
January 19583. Immediately, a vast range of potential applications
was possible that had been the dream of scientists and
science-fiction writers for years. Many ideas were pursued that were
of immediate use to the military. Those that have found the most
success can be broadly grouped into systems that gather information
and those that relay it.
The first class of information-gathering systems includes weather
satellites that image the earth in various spectral bands to
determine cloud cover, moisture content, and related information.
These systems include both geosynchronous satellites that remain
relatively stationary over a region of the earth and low orbiting
systems like the Defense Meteorological Satellite Program (DMSP)
satellites that gather more highly resolved data for the military
operator. Also, earth resources satellites like Landsat have imaged
the earth in various spectral bands to determine such information as
crop usage, environmental changes, and shifts in the rural/urban mix
of the population.
Both weather and resources satellites exploit the
visible and infrared regions of the electromagnetic (EM) spectrum,
passively collecting the data and then sending it to ground stations
via radio and microwave links. Multispectral imaging (MSI) systems
have been developed to collect data at different wavelengths
simultaneously providing much more information. The MSI systems can
determine such information as the health of forests and crops. They
can also differentiate between wet and dry ground and the
composition of structures, providing measurement and signature
intelligence (MASINT) to the military users. Image Intelligence (IMINT)
gathering from orbital platforms began early with the Corona
program, a recently declassified imaging satellite system.4
Most of
these space assets are still cloaked in secrecy, but clearly the
information is collected across a wide range of the EM spectrum,
including radio and microwave transmissions as well as visible and
infrared images. Some of the systems are passive, collecting the
signals with extremely sensitive antennas and optical receivers,
while others are active, using radar to penetrate cloud cover and
observe targets on the ground. Other passive imaging systems have
been placed in orbit to detect missile launches that might signal a
hostile act against the US or its allies. For example, the Defense
Support Program (DSP) surveillance satellites provided launch
detection of Scud missiles during the Gulf War that permitted impact
point prediction and early warning.5 With the exception of
space-based imaging radar satellites, all of these satellites are
passive collectors of information. The laser, as discussed later,
offers unique advantages in gathering information by actively
illuminating targets.
The second class of information-relaying systems transfers voice,
data and image information, and encompasses numerous commercial
systems as well as dedicated military systems. For example,
communications satellites typically use microwave frequencies to
carry the information from the earth to the satellite, between
satellites, and back to the earth. The Global Positioning System
(GPS) provides a second example, relying on a constellation of
satellites to generate and transmit highly accurate time signals for
precisely determining location. These guidance or navigation systems
offer incredible capabilities that are beginning to revolutionize
military and civilian travel on or near the surface of the earth. In
this second class of information-relaying systems, the laser offers
unique capabilities for extremely high data rates and highly
accurate guidance systems.
To date, no weapon systems have been stationed in space, primarily
due to technological and treaty limitations. Both the Soviet Union
and the United States have conducted tests of anti-satellite systems
using various types of kinetic and chemical energy warheads to
intercept and destroy orbiting platforms. The use of directed energy
weapons (DEW) such as lasers, high power microwaves (HPM) or charged
particle beams (CPB) has been considered in great detail by such
programs as the US Strategic Defense Initiative (SDI). Some
space-based DEW components, such as the ALPHA laser, have been
constructed and tested on the ground, but no systems have been
tested in orbit. Without question, space-based lasers could be
fielded in 10 to 20 years that can destroy targets in space as well
as on or near the earth’s surface. The challenges involve
engineering and cost, rather than the fundamental laws of physics.
Treaties, such as the Outer Space Treaty of 1967 and the
Anti-Ballistic Missile (ABM) Treaty, restrict the United States from
placing certain types of weapons in space and need to be carefully
considered as lasers move into the space environment. Other pending
international agreements such as the “Blinding Laser” ban could
affect space-based lasers even though space-based lasers would not
be specifically designed for blinding personnel.6 Technologists and
operational commanders need to be aware of the political issues that
can radically alter the new systems under development.
Military Uses of Lasers
For many years, the laser was touted as a “solution in search of a
problem,” as most of the early applications remained in the research
laboratory.7 In the past twenty years, lasers have solved myriad
problems. The advances in laser technology have truly revolutionized
a variety of areas, including medicine, telecommunications,
industrial welding and cutting, and data processing. The ubiquitous
laser bar code scanners and compact disc players provide almost
daily contact with lasers for most people in the developed world.
The military was one of the first services to see the potential for
lasers in many applications. The early hopes of fielding a
high-energy laser (HEL) weapon have yet to be fully realized by the
United States, although the technology is well in hand. The AF’s
Airborne Laser (ABL) program aims to field an operational HEL to
negate theater ballistic missiles in their boost phase by early in
the 21st century.8
The laser was quickly employed as an aid to other weapon systems.
Innovative scientists and engineers used the beam to point at a
target and generate an aim-point that could be used to guide a bomb
precisely to the target.9 As a type of precision guided munitions (PGM),
the PAVE (“Precision Avionics Vectoring Equipment”) series of laser
target designators (LTD) and the associated PAVEWAY laser-guided
bombs (LGB) have been tremendously useful in conflicts from the
Vietnam War to the Gulf War. Fielded military laser systems also
include highly accurate range-finders and secure communication
systems. Lasers have also been very useful for training aids such as
the MILES system, the military equivalent of “laser tag” available
now to the general public. Recently, laser spotlights have provided
both visible and infrared illumination for improved use of night
vision devices (NVD). As widespread as the laser has become in the
US military, it has yet to be effectively employed in space. It is
this shortcoming that motivates this study.
Military Use of Lasers in Space
This study begins with a brief discussion of the exploitable
characteristics of both the space environment and the laser to
create the backdrop for the subsequent analysis of various concepts
for lasers in space. After defining a taxonomy for space-based laser
systems and relating it to the operational concerns, the study
examines several recent strategic studies with respect to how
different groups of analysts envisioned using lasers in space for
military purposes.
These concepts are scored with a simple set of
technological and operational criteria in order to determine the
most attractive near-term concepts for technology demonstrations.
Back to Contents
II. EXPLOITABLE CHARACTERISTICS OF SPACE
The region outside the atmosphere offers a unique environment for
military operations.
The ability to exploit the characteristics of
space will give the warfighter a competitive edge in virtually all
conflicts. Highlighting the differences between “air” and “space” in
terms of doctrinal development, one study identifies the three
characteristics of space systems — emplacement, pervasiveness, and
timeliness — that benefit from the features of space.10 A brief
review of these features provides background to understanding how
space-based laser systems can be most effectively deployed.
A slightly different phrasing of these characteristics is given in
an Air Force Space Command publication:
The space high ground offers tremendous advantages not found on
Earth. Space allows countries like the United States to watch the
entire globe on an almost “real time” basis [timeliness]—getting
information nearly the instant it’s needed. Space assets are always
available [emplacement] when America needs them. Space also gives
military planners the added advantage of seeing the entire
battlefield—the highest possible vantage point [pervasiveness]
allows friendly forces to watch over an enemy, serving as both a
watchdog and a deterrent. Space systems also offer the distinct
advantage of longevity. Aircraft missions last a few hours, while
satellites are constantly operational for years.11 [Emphasis added.]
While these unique characteristics of space are only beginning to be
exploited effectively, an important caveat is that space is an
international environment over which no nation has sovereign
control. The fact that space systems do not need basing rights or
over-flight approval increases the freedom to conduct operations,
but this liberty not only creates vulnerabilities but increases the
possibility of conflict with nations that attempt to interfere with
the space systems of other nations.
Emplacement
Emplacement means that space systems can be pre-positioned in orbits
which offer optimal support when needed. This characteristic might
also be called persistence or presence. With proper ground support
systems and sufficient satellites, space systems can be maintained
in a state of wartime readiness, and thus are “inherently ready to
support military operations at all times, which avoids the potential
complications of basing rights and over-flight permission.”12 Given
the remoteness of space as well as the difficulty and expense of
deploying systems there, the characteristic of emplacement demands
foresight by planners so that these systems can be pre-positioned to
ensure availability in a crisis.
Pervasiveness
Outer space begins between 50 to 100 miles above the earth’s
surface, depending on the criteria used.13 This region surrounds the
earth’s surface and thus permits a presence over all land, sea, and
air targets. Surveillance systems positioned in space reduce the
risk of being surprised and complicate a potential adversary’s
ability to hide. Systems in low earth orbit (LEO) move at high
velocities, traveling over 7 kilometers every second and completing
an orbit every one to two hours, depending on altitude. LEO
satellites give the best resolution due to their proximity to earth,
but more satellites are required to permit continuous coverage of
various ground and sea locations.
Systems in middle earth orbit (MEO)
have longer orbital periods, like the 12-hour period for the
semi-synchronous orbits of the GPS satellites. This means that fewer
satellites are required—only 24 satellites are needed for GPS to
provide adequate coverage for global navigation. Satellites in
geosynchronous earth orbit (GEO) have a 24-hour period and remain
roughly over the same point on the earth’s surface. With just three
satellites, the entire surface of the earth can be covered, with the
exception of the higher latitudes. However, due to the greatly
increased altitude (22,300 nautical miles (NM)), achieving high
resolution with GEO systems (e.g., the ability to discern or point
at small targets) is challenging.
Timeliness
Depending on the type of orbit, a sufficient number of space systems
can provide near-instantaneous coverage of every point on the globe,
at the cost of increased complexity in controlling the network.
Because the network is linked by EM radiation that travels at the
speed of light (186,000 miles per second), a properly fielded space
system permits “near-real-time transfer of information” to
war-fighters and facilitates “rapid application of force” against
almost any type of target.14 Also, the high orbital speeds mean that
space systems are frequently overhead, which translates into more
engagement opportunities for the military commander.
Unattenuated Propagation of Electromagnetic Radiation
A vacuum is the ideal environment for propagating electromagnetic
radiation. Any intervening medium, such as air, bends, scatters or
absorbs the radiation, depending on the frequency or wavelength of
the radiation and the type of medium. In the near vacuum of space,
all frequencies propagate with essentially the same low attenuation.15
For space-based systems that transmit EM radiation toward the earth,
how far this radiation penetrates into the atmosphere depends
strongly on the wavelength due to both absorption and scattering.
For example, clouds attenuate visible light much more than microwave
frequencies used in radar systems. Thus, radar gives better
all-weather coverage than lasers when propagating through the air.
From space, radar systems are able to penetrate some clouds to image
objects on the ground when optical imaging systems cannot discern
the target through cloud cover. Many optical wavelengths do
penetrate through clear air with low absorption, allowing
space-based lasers to focus optical energy on targets at or near the
earth’s surface for applications such as illumination,
communication, remote sensing, target designation, or
target
destruction.
By virtue of the negligible attenuation of EM radiation in space and
the short wavelengths of lasers, laser beams can propagate for
extremely long distances with relatively little growth in the
cross-sectional area of the beam. This helps maintain the energy
density in the beam, meaning that more energy can be delivered to a
target, both cooperative ones like communications satellites and
uncooperative ones like reentry vehicles.
Challenges
The characteristics of space pose many challenges to both systems
and people who transit through space. A brief discussion of a few of
these challenges will support the subsequent discussion of laser
systems that must operate in space for long periods.
Environmental Hazards
Ambient ionizing radiation poses unique risks
to systems deployed in space. The atmosphere filters out a wide
range of threats to people and systems near the surface. Ionizing
radiation means any radiation that has sufficient energy to knock
electrons out of their orbits around the atomic nucleus. It includes
very short wavelength EM radiation, such as extreme ultraviolet and
x-rays and energetic particles consisting primarily of protons,
alpha particles, and electrons. Much of the ionizing radiation comes
from the sun. Very high-speed particles, cosmic rays, come from
other sources. This naturally occurring radiation can destroy
electronics, cause software errors by changing memory values, and
degrade hardware.
While the EM radiation from the sun is fairly
constant, the intensity of particles varies greatly with time and
peaks with solar flares. The particles interfere with satellite
operations and can disable satellites. Forecasting the space
environment is an emerging area of meteorology. Thus, space systems
that must remain in orbit for many years can face a large cumulative
exposure as well as brief, high doses. Shielding can reduce the
effects of ionizing radiation but at the expense of increased
weight. Careful selection of materials can also reduce the
deleterious effects. For this reason, extensive radiation hardening
programs for critical components are underway within NASA and
DOD.
A further complication is the existence of the Van Allen radiation
belts, which are regions of high-energy particles trapped in the
earth’s magnetic field. Primarily consisting of protons and
electrons, these particles spiral around the magnetic fields,
reflecting back and forth between the earth’s magnetic poles, where
the magnetic flux becomes more concentrated. The interaction of this
radiation with the air gives rise to the beautiful aurora borealis
and aurora australis. There are two Van Allen belts. The inner belt
begins at an altitude between 250 and 750 miles (depending on
latitude), extends to about 6,200 miles and covers from about 45
degrees north to 45 degrees south in latitude; the outer belt begins
at about 6,200 miles and extends out to as much as 52,000 miles.16
Due to orbital constraints most space systems will operate within
one of these two belts. Spacecraft in LEO appear to receive an
insignificant amount of radiation from the Van Allen zones, while
spacecraft in MEO or GEO may pick up substantial doses.17
Another challenge posed by space is extreme temperatures. With no
atmosphere to help conduct heat, systems can become either extremely
hot or extremely cold, depending on exposure to the sun. As a
spacecraft orbits, it moves into the earth’s shadow where the sun’s
heating is eliminated and radiative cooling occurs. When it again
enters the sunlight, the heat builds up on the surfaces exposed to
the sun. Such thermal cycling causes expansion and contraction of
materials and needs to be considered in the design of spacecraft.
The cycling may eventually cause cumulative damage to the spacecraft
and could cause the misalignment of sensitive optical systems.
Cost
Currently, people launch objects into space by using chemical
rockets to give the object sufficient altitude and velocity to
attain orbit. The costs of building and launching the space boosters
include both the cost of the booster and the cost of the facilities
and manpower to accomplish the task. Current costs of orbiting a LEO
satellite are very high, ranging from $3,000 to $10,000 per pound.18
The Space Shuttle payloads cost roughly $8,000 to $9,000 per pound
to launch.19 Several programs are underway within NASA and DOD to
explore ways of bringing the cost down below $1,000 per pound. These
programs include reusable chemical rocket systems, such as the
single-stage-to-orbit (SSTO) concept tested in the Delta Clipper
rocket; a reusable space plane that would take off and land like a
conventional aircraft; and novel ideas, such as electromagnetic rail
guns and air guns to put satellites in orbit without chemical
rockets. The relevant point for this discussion is that because cost
determines the feasibility of putting systems in space, anything
that can be done to reduce weight is highly valued. As will be
discussed later, laser systems may offer significant reductions in
weight while maintaining or expanding system capabilities (such as
communication bandwidth).
Manpower-Intensive Operations
At present, each US space launch
requires large teams of highly trained personnel to design, build,
launch, and operate space systems. The launch facilities are
expensive, as are the global network of ground stations that are
currently necessary to maintain operational control over the
spacecraft. Research is underway to make spacecraft more modular and
standardized, which would reduce design and manufacturing costs.
Advances in software and increasing space infrastructure (such as
GPS as a navigational grid) will make spacecraft more autonomous and
reduce the need for large numbers of highly skilled engineers to
operate the systems. Manpower reductions should be included in the
design of all space systems.
Self-Protection
Systems placed in space face both accidental and
intentional threats. As more satellites have been launched, the
amount of man-made debris (“space junk”) has increased. The Air
Force currently tracks 8,168 objects in orbit, only 7 percent of
which are active satellites.20 When combined with naturally
occurring meteorites, the risk of colliding with a hypervelocity
projectile is not insignificant, particularly if spacecraft remain
in orbit for years. Proper orbit selection, additional shielding, or
maneuvering are options to increase the spacecraft’s survivability.
Since from 1950s, military researchers have considered ways to
destroy satellites.21 Anti-satellite (ASAT) weapons using nuclear
warheads, conventional explosives and hypervelocity kinetic kill
warheads as various ways to destroy a satellite were tested by the
United States and the Soviet Union. There were suspicions that
Soviets blinded optical sensors on US early warning satellites in
the mid-1970s but, even if true, the laser systems would likely have
been R&D prototypes rather than fielded hardware.22 Of course, a
successful R&D system leaves a residual operational legacy, as the
Russians may possess at their laser research facility in Sary Shagan,
which is located in Kazakstan.23 The US Air Force has an on-going
ground-based laser anti-satellite (GBL ASAT) research program.
Certainly directed energy systems such as lasers or high power
microwave (HPM) weapons could be used to disable or negate
satellites, but they have not been fielded at this time due to
substantial technical challenges and treaty agreements. At present,
only a residual capability exists within the United States or Russia
for ASAT missions. However, the predictability of orbits and the
remoteness of space complicate the ability to protect spacecraft
from attack.
Back to Contents
III. EXPLOITABLE CHARACTERISTICS OF LASERS
The laser is a unique source of optical radiation which has a number
of characteristics that can be exploited for military space systems.
As a number of these applications carry over to the civilian market,
NASA and the private sector are interested in exploiting the
laser for space applications. There are a number of sources that
describe the basics of lasers.24
Briefly, the laser uses the phenomenon of stimulated emission to
generate a very narrow beam of light that is usually highly
monochromatic. Such a beam has a high degree of coherence as
compared to other optical sources like the sun or incandescent light
bulbs. The coherence permits the beam to propagate long distances
with little spreading and to be focused onto a small area. Within
the ever-expanding variety of types of lasers, with different
wavelengths, power levels, temporal characteristics and operating
efficiencies, the unifying characteristic is the generation of
coherent radiation.
In analyzing any laser application, it is convenient to break the
process into three parts:
The laser system can be further divided into the laser,
where the coherent radiation is generated, and the optical system
that takes the beam from the laser to the output aperture where the
beam enters the propagation medium. At the target, the light from
the laser must be absorbed to cause an effect, whether that effect
is an electrical response in a detector or a destructive effect for
a weapons application. Each part (the laser system, the propagation
medium, and the target) plays an important role in evaluating the
feasibility of a laser application. Keeping this taxonomy in mind
will help avoid fixating on any single part to the exclusion of the
others.
Directionality
One of the key properties of lasers is that the output beam is
highly directional. Typical laser beams have a divergence of less
than a milliradian,* and some systems can be designed to have sub-microradian
divergences. Because of their small size, semiconductor diode lasers
usually have divergences measured in degrees, expanding rapidly.
However, this beam divergence can be substantially reduced by using
external optics. A laser system with an output beam diameter of one
meter could readily have a 0.05 milliradian beam divergence,
expanding to only about 25 meters after traveling 500 kilometers.
This pencil-like beam of light permits highly accurate placement of
energy on a target for a variety of applications such as target
designation and efficient communication links. Additionally, the
beam can be used for covert applications because it is very
difficult to detect the beam without intercepting it. The
disadvantage, of course, is that pointing the beam requires a high
degree of precision.
Wavelength, Bandwidth, and Tunability
A laser operates in the infrared, visible and ultraviolet regions of
the electromagnetic spectrum, from one millimeter to 100 nanometers
in wavelength. Typically, lasers are described by their wavelength
(l) as contrasted with radar systems that are characterized by
frequency, because the laser’s frequency is from 10,000 to 1,000,000
times higher than typical microwave radars. Both microns (mm or 10-6
meters) and nanometers (nm or 10-9 meters) will be used in this
study to characterize lasers. Radar systems usually have wavelengths
on the order of millimeters to centimeters. Many lasers generate
light in a very narrow band around a single, central wavelength.
Because this characteristic manifests itself in visible lasers as a
very pure, single color, the narrow linewidth is termed
monochromaticity. For example, the neodymium laser used in most
laser designators (the ubiquitous “Nd:YAG”) generates an output beam
at 1.064 microns, with a typical bandwidth of 0.00045 microns, an
amazingly narrow linewidth of 0.04 percent of the central
wavelength. This spectrally pure output is critical for a multitude
of applications, including remote sensing for specific chemical
constituents and high signal-to-noise ratio (SNR) communications.
Some types of lasers operate on several different wavelengths
simultaneously, such as the argon ion laser that emits most of its
light at 488 nm and 514.5 nm. Multiline emission can be both a
benefit and a detriment, depending on the application.
While most lasers will only operate on discrete wavelengths, some
types can be tuned over a range of wavelengths, giving an additional
agility that has multiple uses. Examples of tunable lasers include,
-
the titanium sapphire (Ti:S) laser
-
the chromium:LiSAF laser (where
the host material is a crystal of LiSrAlF6)
-
the chromium:LiCAF
lasers (where the host material is a crystal of LiCaAlF6)
These
three lasers are solid state systems that have great potential for
space applications, such as remote sensing of the atmosphere from
orbit. They also have the added potential of being pumped by diode
lasers or other solid state lasers that are diode-pumped. Thus,
all-solid-state systems can be constructed with much improved
reliability and durability.
Table 1 shows the tuning range of these
three lasers.
Table 1. Typical Tunable Lasers25
Laser Type |
Lasing Ion |
Wavelength Range |
Titanium Sapphire |
Ti3+
|
660
to 1180 nm |
Chromium LiSAF |
Cr3+
|
780
to 920 nm |
Chromium LiCAF |
Cr3+
|
720
to 840 nm |
|
As discussed in more detail in Appendix B, some special types of
materials respond nonlinearly to light passing through them and can
generate new wavelengths of light.
Such nonlinear optical (NLO)
materials are the subject of contemporary research. The most common
is the frequency doubling crystals that cut the wavelength in half,
so that the infrared emission of a Nd:YAG laser (at 1064 nm) can be
converted into a visible beam (at 532 nm). Frequency doubling can be
fairly efficient, with reported values of 50 to 80 percent
conversion from the fundamental wavelength to the doubled
wavelength. Other nonlinear systems, like optical parametric
oscillators (OPO), can generate a tunable output.
While the
technical details of such systems are beyond the scope of this
study, they highlight the possibility of “wavelength agility” or the
ability to tune the output wavelength of the laser. However, at this
time, only a limited number of NLO materials are available. The
efficiency at which they operate tends to be low. Also, obtaining
efficient nonlinear effects requires high peak powers from the laser
beam, which can damage the NLO material. The threshold at which NLO
materials are damaged is usually low, making scaling to systems with
high average power challenging. Research in material science is
likely to push back these limits.
Another NLO effect that can be used for wavelength shifting is
stimulated Raman scattering (SRS). Raman scattering occurs when a
beam of light passes through a material and excites a very weak
transition within the material, leaving some of its energy. The
emitted light is shifted to a longer wavelength. If the process is
stimulated in a method analogous to the operation of a laser, a
significant amount of the light can be shifted to the new
wavelength. SRS is a complicated process beyond the scope of this
study but offers great potential for laser systems.
The narrow bandwidth of the typical laser can be reduced even
further by specialized design of the laser. If this narrow beam is
reflected off a moving object, the frequency of the reflection will
be shifted slightly by the Doppler effect, permitting direct
measurement of the velocity of the object. The “object” can even be
a region of air, allowing direct, remote measurement of wind speed.
Temporal Modulation
The output from the laser can be either continuous (called
“continuous wave” or CW) or pulsed. Usually a laser is called CW if
the output lasts more than 0.25 second.26 A pulsed laser is
characterized by the pulse duration, which is measured in seconds.
If the laser is repetitively pulsed, the pulse repetition frequency
(known as the prf and measured in Hertz) is the period from the
beginning of one pulse to the beginning of the next pulse. The duty
cycle of the laser is the product of the pulse duration and the prf
and gives a measure of what percent of the time a laser is emitting.
A duty cycle of 50 percent means the laser is emitting energy half
of the time.
Most lasers of interest to the military are either CW or have very
short pulses on the order of nanoseconds. For example, the laser
being developed for theater missile defense in the Airborne Laser is
a CW laser. In contrast, the typical laser designator emits pulses
of about 10 nanoseconds in duration, with a prf of 10 Hz, giving a
duty cycle of 0.0000001. Some lasers use much higher repetition
rates, on the order of kilohertz or higher, such as semiconductor or
diode lasers that have great potential for communication systems.
Imaging laser radars under development may use pulses with durations
of less than a nanosecond in order to achieve good spatial
resolution. (Light moves about 1 foot in a nanosecond, so temporally
resolving the reflections of pulses off a surface can give detailed
three-dimensional information if the pulses are short enough.)
Output Power and Energy
The laser beam contains energy in the form of electromagnetic
radiation that travels at the speed of light and has no mass. CW
output is usually characterized by the power in the beam measured in
Watts, while pulsed output is characterized by the energy in each
pulse, in Joules. Repetitively pulsed systems are also characterized
by their average power. The range of output power from useful CW
lasers ranges from milliwatts to hundreds of kilowatts. Megawatt
lasers are feasible but pose unique challenges when scaling the
output power to that level. The MIRACL laser system is a
megawatt-class laser at the High Energy Laser System Test Facility
on White Sands Missile Range that is routinely used for damage
testing and other studies. Commercial users can even buy time on
this device for their own testing.27
All of the laser power is concentrated in a small solid angle due to
the narrow beam. This means that even small lasers, like the helium
neon (He-Ne) lasers frequently used as pointers, have output beams
that are brighter than the sun. Here the term brightness is
rigorously used to mean the amount of power being emitted per unit
area of the source per solid angle.
A laser is classified as a severe hazard (denoted Class IV by
federal regulations) if the output power exceeds 0.5 watts—quite a
contrast with a 100-watt lightbulb that emits its energy in all
directions. The reason is the high brightness of these lasers. Class
IV lasers, the most dangerous category, are sufficiently intense
that the direct beam is a hazard to both eyes and skin, causing
nearly instantaneous injury. The beam could also ignite some objects
that it strikes. (Several fatalities have been reported due to
laser-generated fires.) The Class IV laser is so dangerous that even
the diffuse reflection of the beam from a wall might cause eye
injury. Stringent control measures are required for the use of these
lasers, even though the output power may appear to be low.
To give a feeling for these numbers, the damage threshold for “soft”
targets like paper or skin is roughly one Joule per square
centimeter, assuming a one-second exposure. Wood surfaces are
damaged at approximately 10 J/cm2 while metal surfaces are damaged
in the range of 100 J/cm2.28
Note that these numbers are just order of magnitude values to
illustrate the concentrated power of the laser because actual damage
thresholds depend on a number of factors. For example, the New World
Vistas study by the AF Scientific Advisory Board cited a damage
threshold for Scud missiles as roughly 1,000 J/cm2 as a first-order
design parameter.29
Pulsed lasers emit light in short bursts. The energy per pulse for
useful lasers begins in the millijoule range and reaches into
kilojoules. A typical laser designator, like the LANTIRN system used
by the AF, emits very short pulses (of about 10 nanoseconds in
duration) that contain over 100 millijoules of energy. Assuming a
square pulse, these pulses have a peak power of 10 megawatts
(dividing 100 millijoules by 10 nanoseconds). It is the high peak
powers in pulsed lasers that can be exploited for a variety of
applications. If the output of even the modest LANTIRN is focused in
the air, it can be sufficiently intense to ionize the air in a
phenomenon known as air breakdown.
“Deep Magazine”
The amount of power or energy depends strongly on the application.
For communication systems, the use of sensitive detectors can permit
the use of low energy pulses and lasers with average powers of a few
watts. The operational lifetime of such systems could easily be
decades. For weapons applications, megawatts or tens of kilojoules
can be required to achieve structural damage on distant targets. By
carrying an adequate fuel supply or by using energy from the sun
either directly or indirectly to power the laser, many firings of
the high-energy laser can be possible, thus giving a “deep magazine”
when compared to conventional systems. Estimating the energy
requirements is one of the key assessments of the laser concepts to
be discussed later in order to appraise the feasibility of the
concept.
Adjustable “Power on Target”
The ability to adjust the energy output of a laser system is also an
exploitable characteristic.30 A single, properly designed system
could be used in a passive mode (no output beam) for surveillance,
in a low power mode for target illumination for active imaging and
for target designation for PGM delivery, and in a high power mode
for negating a target.31
No Recoil from Light Beams
One unique aspect of directed energy beams like lasers is that there
is no recoil in the weapon due to the output beam, as there is with
kinetic energy weapons. This means that there is negligible effect
on the orbital parameters in even a HEL due to the beam. However,
there can be substantial thrust generated by the exhaust gases from
a high-energy, chemical laser as it creates the output beam. The SBL
systems, such as the Alpha laser, are designed to reduce the impact
on the orbit of the space-based laser.
Speed of Light Delivery
Laser beams travel at about 300,000 km per second, or about Mach
1,000,000.32 This permits near-instantaneous engagement of targets,
even for targets at very long distances. This greatly reduces the
need to lead the target as compared to kinetic energy weapons like
missiles. (There may still a need for a significant point-ahead
angle if the relative velocities between the laser system and the
target is large, as in the case of pointing a laser at a satellite
from a ground-based laser.) If the target can be tracked visually,
the laser beam can be placed on the target and, if sufficient energy
is delivered, the desired effect can be achieved. This exploitable
characteristic is particularly useful for operations in which time
is critical or the engagement range is extremely long.
Freedom from Newtonian Constraints
Conventional weapons rely on kinetic energy in the form of a
high-speed impact or chemical energy in the form of explosives to
attack targets. These weapons are subject to the Newtonian laws of
physics, such as gravitational attraction and aerodynamic forces.
Gravitational attraction and aerodynamic forces, such as crosswinds,
complicate the targeting by requiring trajectory considerations
during the weapon delivery.
Aerodynamic forces such as drag and lift
affect how much range can be achieved from the weapon. The laser
beam is not significantly affected by gravity unless the propagation
path is extremely long where bending of light in gravitational
fields is significant. Aerodynamic forces do not slow the beam,
although the atmosphere can scatter and absorb the optical radiation
as discussed below. Thus, the laser is free from the usual
constraints of Newtonian physics.
“Ilities”
One topic of constant concern for system developers is the “ilities”
such as reliability, maintainability and affordability. This is
particularly important for space systems because access for repairs
is almost non-existent. The lengthy series of tests to “space
qualify” hardware is one of the factors that drive up the cost of
space systems. Some types of lasers are very reliable, relatively
free of maintenance requirements, and have a long service life. For
example, semiconductor diode lasers are now able to operate for tens
of thousands of hours.
The fact that every compact disk player
contains three diode lasers is quiet testimony to the reliability
and affordability of these types of lasers. Semiconductor lasers are
a leading candidate for some of the space-based applications such as
communication. Also, all-solid-state laser systems are now being
marketed commercially, such as diode-pumped Nd:YAG lasers, that are
very rugged and reliable, require little maintenance, and have a
long service life while generating substantial output power.
Replacing the flashlamps with laser diodes was a key change to this
advancement. Such lasers would be appropriate for applications such
as space-based target designation and remote sensing.
Other systems,
such as some closed-cycle gas lasers that can be scaled to fairly
high powers, can be operated continuously in the laboratory for
thousands of hours. These lasers have potential for laser
illuminators, active imagers, and possibly even weapons. A final
point related to affordability derives from the much shorter
wavelength of lasers as compared to microwave systems. This means
that the size and weight of the spacecraft can probably be reduced
as compared to microwave systems that perform analogous functions,
such as imaging or communication.
Challenges
The laser has a number of unique characteristics that can exploited
for military applications, but there are challenges and inherent
limitations. These limitations can be overcome or ameliorated by
proper design. The challenge to understanding laser devices is to
consider that the entire system, which consists of the laser device
and its supporting systems on a space-based platform, its beam
through space or air to impact on a target of some sort, and its
ability to influence that target in some way. It is essential to
consider the entire laser system, rather than just the laser itself,
in order to identify the limiting technical challenges. In some
concepts, it may be generating sufficient power from the laser
device, as in the case of space-based laser weapons for national
missile defense. In other cases, the prevailing challenge may be the
acquisition, pointing, and tracking (APT) system or propagating the
beam through the atmosphere, as in laser communication systems.
In
still others, the challenge may be in gathering weakly scattered
light from the target in order to gather the desired information, as
in the case of direct wind measurement or remote sensing of
effluents. Finally, the nature of the target may be the limiting
constraint, as each target has its characteristic reflection and
absorption properties. In assessing any concept, the entire
end-to-end system needs to be considered.
Efficiency
The process of generating the highly coherent laser beam
is usually very inefficient.* The Nd:YAG laser is only about one
percent efficient, while the popular helium-neon laser is only about
0.001 percent efficient. The unique features of the output beam make
these inefficiencies bearable. Fortuitously, semiconductor lasers,
which generate light by direct conversion of electrical current to
photons, are very efficient, achieving 20 to 50 percent
efficiencies. At present, these systems do not produce the power
levels necessary for high-energy laser weapons.
But a laser, such as
the carbon dioxide laser (used in the Airborne Laser Laboratory
(ALL) to shoot down several Sidewinder missiles), has an efficiency
on the order of 20 to 30 percent, which can achieve output powers of
hundreds of kilowatts. The chemical efficiency of hydrogen fluoride
(HF) lasers, being considered for space-based laser weapons, can be
up to 20 percent or more, while the electrical efficiencies can
exceed 150 percent, because the energy in the beam comes from a
highly exothermic chemical reaction.33
It is critical to determine where the remaining energy goes, which
inevitably ends up as waste heat and must be removed from the laser
system. In some lasers, like the HF laser, the exhaust gases carry
away the heat. In other lasers, such as the Nd:YAG or semiconductor
laser, some method must be used to extract the heat from the laser,
such as flowing cooled water within the laser. If it is allowed to
remain in the laser, the performance of the laser is likely to be
degraded or, in the extreme, the laser may be damaged. Dissipating
heat in a spacecraft can pose serious problems.
Refueling
Some types of lasers consume fuel while they are
operating. For example, hydrogen fluoride lasers need hydrogen,
fluorine, and carrier gases such as helium. The designs usually
include enough consumables for the expected number of engagements.
However, as scenarios shift and requirements change, the capability
to refuel these types of laser systems through a space
transportation system would be very useful, although quite
expensive.
The difficulty in refueling certain types of lasers makes them less
attractive. It also increases the desirability of other lasers that
obtain their energy from electricity or directly from the sun.
Semiconductor lasers, Nd:YAG lasers, and tunable lasers, such as the
titanium sapphire laser, can be efficiently operated using
electricity. An atomic bromine laser, operating at 2.714 microns,
has the potential of being directly pumped by sunlight without
expending any fuel. This laser is currently under investigation at
the AF Phillips Laboratory.
Target Acquisition
The disadvantage of the small divergence of the
laser beam is the challenge of accurately pointing it at the desired
target, whether that target is a communications satellite or a
nuclear warhead. The basic issue is acquiring the target in order to
know where to point the beam. (This is part of the acquisition,
pointing, and tracking (APT) process that is inherent in many
potential laser applications.) For cooperative targets, such as a
satellite in a communications network, a corner cube reflector could
be used that reflects any incident light directly back at the
source.
By using a laser beam that has a larger divergence,
essentially a laser floodlight, a wider region of space can be
scanned until the retroreflection is sensed and the narrow beam
pointed at the target. For uncooperative targets, other methods of
acquiring the target need to be considered, such as passive optical
sensors and active microwave radar systems. Using the GPS system and
recently-developed inertial reference units, space-based laser
systems will be able to point at targets on or near the surface of
the earth with very high accuracy. Non-mechanical beam steering,
which involves tracking with no moving parts, is also being
developed to permit high speed tracking of targets such as missiles
or satellites.
Propagation into the Atmosphere. Some of the possible applications
of space-based lasers involve aiming the laser back into the
atmosphere. This includes such concepts as remote sensing of
chemical effluents, measuring wind speeds, and negating enemy
targets on the ground, on the sea, or in the air. However, the
atmosphere attenuates many wavelengths, which greatly reduces the
amount of energy that can be put on the target. For example, the
leading candidate for a space-based laser weapon is the TRW’s
hydrogen fluoride Alpha laser, which lases at 2.7 microns, a
wavelength that is strongly absorbed by water vapor in the air.
Thus, this laser could only attack targets at or above 30,000 feet.34
Visible wavelengths penetrate far deeper into the air, but
another phenomenon that attenuates light is Rayleigh scattering that
increases as the fourth power of frequency. This means that shorter
wavelength beams will scatter more significantly than infrared
beams, reducing the energy that reaches the target and increasing
the size of the laser beam. The optimum wavelengths appear, at first
order analysis, to be the near infrared regions of 0.7 to 1.4
microns. Fortunately, a large number of lasers exist in this region,
although few that are scalable to high powers. For example, the
laser for the Airborne Laser is a chemical oxygen-iodine laser
(COIL) that operates at 1.315 microns in this preferred region.
Target Coupling
Another challenge to using lasers in space is to
determine the most efficient way to couple the laser energy onto the
target. In order to cause an effect, the laser energy must be
absorbed by the target. For cooperative targets, this means finding
a suitable detector that has a good response to the particular
wavelength. Detector materials are fairly mature and improving, so
this is unlikely to limit laser applications. However, for
uncooperative targets like warheads, the laser radiation is usually
absorbed at or near the surface of the target. Some of the light
will be reflected as well. Thus, the laser beam will have to work
its way into the target in order to cause structural damage. For
fairly “soft” targets like satellites, this may not be
difficult—parts like solar panels and optical systems can be readily
damaged. But burning through a reentry vehicle’s outer skin would be
very difficult.
Laser Safety
Laser beams can injure people, particularly if a
person looks directly into the beam of a visible or near infrared
laser with wavelengths from 400 to 1400 nm.35 Even a millijoule
entering the cornea can cause a catastrophic hemorrhage of the
retina, because the optics of the eye increase the intensity of the
laser beam by reducing the size of the beam by about a factor of
100,000 as the light travels from the cornea to the retina. Retinal
injuries are permanent, leading to some loss of sight. Even Class
IIIa lasers with output powers in the 1 to 5 milliwatt range can
burn the retina. Thus, space-based lasers in this wavelength range
could pose a safety hazard if pointed toward the earth.
Unfortunately, the term “eye-safe” has recently become popular when
referring to lasers that emit wavelengths longer than 1400 nm, even
if the output power or pulse energy puts the laser in Class IV as a
severe hazard. The notion is that the retina would be safe and that
the cornea might be injured but would heal. This ignores the reality
that deep cornea burns are permanent, although corneal transplants
are possible. Some people are even referring to deuterium fluoride
HEL weapon prototypes (operating at 3.8 microns) and 50 watt carbon
dioxide laser radars (operating at 10.6 microns) as “eye-safe” given
the operating wavelength, even though this ignores the high power
output. Such systems are certainly not eye-safe in any aspect, and
the term sends the wrong message to those not well versed in laser
safety.
Almost all the risks with lasers can be avoided by carefully
controlling where the laser is pointing before it is activated. A
thorough laser safety analysis should be included early in any laser
program, particularly if the laser has new or unusual output
characteristics, such as extremely short pulses with high peak
powers. The biological database that is used to set safety standards
may not be appropriate for some new types of lasers, and gathering
new data to set new safety thresholds is time-consuming, challenging
research. Thus, the program manager must assess the safety risk
early in coordination with DOD laser safety experts.
Back to Contents
|