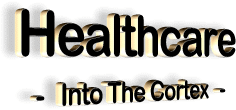
by Clive Cookson
July 31, 2012
from
FT
Website
Scientific advances on the brain
promise to transform the pharmaceutical
industry
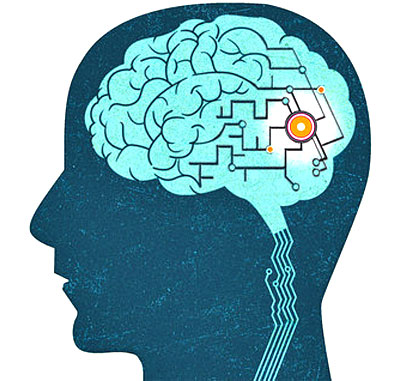
Imagine a
the pharmaceutical companies 20 or
30 years from now.
Moving beyond conventional drugs that
interact biochemically with the body, it will have built a big
“bioelectronics” business that treats disease through electrical
signaling in the brain and elsewhere.
Neurological problems, from stroke and epilepsy to depression, will
be treated through electronic implants into the brain rather than
pills or injections. Even diabetes and obesity will be attacked in
ways that seem like science fiction today, by sending electrical
signals to malfunctioning cells.
That remarkable vision comes from one of the world’s most powerful
pharmaceutical executives, Moncef Slaoui, head of research
and development at GlaxoSmithKline, the British drug company.
“At present every pharmaceutical and
biotech company makes chemical or biological molecules that
target [biochemical] structures such as proteins in the body,”
said Mr Slaoui.
“No one is designing medicines to
interact with the electrical signals that are the other language
of biology.”
With enthusiastic support from Sir
Andrew Witty, his chief executive, and other senior colleagues,
Mr Slaoui wants GSK to become the first large company to make
bioelectronics a central plank of its long-term planning.
“The sciences that underpin
bioelectronics are proceeding at an amazing pace at academic
centers around the world but it is all happening in separate
places,” he says.
“The challenge is to integrate the
work - in brain-computer interfaces, materials science,
nanotechnology, micro-power generation - to provide therapeutic
benefit.”
This phenomenon is in its infancy.
Although GSK appears to be ahead of the
competition in preparing for this transformation of the industry, it
is not yet ready to pump large amounts of direct funding into
bioelectronics. Instead, the company is preparing to play a
coordinating role. That will involve setting up a bioelectronics
institute, offering a large prize for innovation, and pulling in
funds from endowments and venture capitalists.
Work is currently mainly conducted in universities and
commercialization remains extremely limited.
Today, only a minuscule fraction of the electrical activity in
people’s brains can be read by computer. Even so, recent experiments
have given spectacular results.
Optogenetics - The arrival of remote control
for the brain
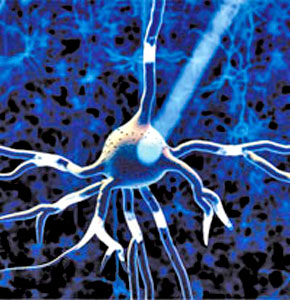
A photosensitive
neuron
Optogenetics - a technology
developed in the past decade - enables neuroscientists to
manipulate brain activity with light.
It has become an invaluable tool for
investigating the roles of different types of neuron, or nerve
cell, in experimental animals and could eventually be used to
treat human diseases.
It has taken brain biology by storm.
“Ontogenetic tools are now
in use by many hundreds of neuroscience and biology labs around
the world,” says Ed Boyden of the Massachusetts Institute of
Technology, who has played an important role in its development.
“We and others are discovering the specific functions of
specific groups of neurons with more precision than is possible
in any other way.”
The technique involves the genetic modification of neurons to
make them produce opsins - light-sensitive proteins that are
normally made in photoreceptor cells in the eye and in some
micro-organisms.
These ontogenetic neurons can then
be switched on and off with different light signals, making
brain activity controllable.
“Optogenetics is a form of
wireless communication in which nerve cells in the brain are
programmed genetically so that you can control their
electrical activity with an optical remote control,” says
Gero Miesenböck of Oxford University, another pioneer of the
field.
Studies were carried out first in
cultures of human neurons and in simple organisms such as
roundworms and fruit flies, and then in mice and monkeys.
“What optogenetics can help us
do is to pinpoint the cells that are causally responsible
for our behaviour,” Prof Miesenböck says.
Last week, Prof Boyden and
colleagues published in the journal Current Biology the first
evidence of changing the behaviour of monkeys - the way the
animals move their eyes - through optogenetics.
Though the technique is not yet ready for clinical use, experts
see great promise in the long term. “There could be the
possibility of using ontogenetic manipulations directly in
humans, in order to restore neural signals that have been
corrupted or lost because of injury or disease,” says Prof
Miesenböck.
Human optogenetics will require two operations. First the
light-sensitive genes are introduced to the patient’s neurons -
probably carried in a harmless virus, as with other applications
of gene therapy. Later a fine fibre-optic cable is inserted
through a small hole drilled in the skull to illuminate the
target area of the brain.
A likely target is Parkinson’s disease, where optogenetics could
provide much more precise therapy than the electrodes that are
today inserted into patients’ heads to provide deep brain
stimulation.
Perhaps the most remarkable was a recent
US clinical trial in which tetraplegic patients
used their thoughts to direct robotic arms.
The research team, led by John
Donoghue and Leigh Hochberg at Brown University, released a
video of a 58-year-old patient called Cathy, whose mental activity
drove a robot to pick up her drinking bottle from a nearby table and
move it up to her mouth so that she could sip some coffee.
A
heartwarming smile illuminated Cathy’s face after she had drunk
without help from a human carer for the first time in 15 years.
The BrainGate system developed by the Brown University team
has a sensor, about the size of a small aspirin pill, with 100
hair-thin electrodes implanted into the top of the patient’s motor
cortex, the brain area that controls movement. It is connected to an
external computer, which translates the neural activity into
commands to drive a robot.
According to another paralyzed patient called Bob, hard thinking is
not required:
“I just imagined moving my own arm
and the [robotic] arm moved where I wanted it to go.”
Still, there will never be a mass market
for personal robots - or even prosthetic limbs - controlled by
tetraplegics. So Prof Donoghue and his colleagues are
working on a second generation system, BrainGate 2.
This would send
signals from the brain to a Functional Electrical Stimulation (FES)
device, bypassing the injury that blocks the natural transmission of
nerve signals and stimulating the patient’s own paralyzed muscles to
contract on demand.
“The ultimate aim is for people who
were paralyzed to walk around in a way that is indistinguishable
from other people,” Prof Donoghue says. “But to get there we
must remember that walking is not only about moving your legs
but also about balancing.”
This example illustrates how advances in
the motor control would have to operate in parallel with work on
other parts of the body that help balance, such as the inner ear.
For many applications, invasive interfaces such as BrainGate, which
are fitted surgically into the brain, may not be appropriate.
Other
researchers are working with electroencephalography (EEG), which
detects electrical activity noninvasively through electrodes, placed
in mesh-like caps on the outside of the skull.
The École Polytechnique Fédérale de Lausanne in Switzerland
runs one of the world’s most advanced brain computer interface
programs using EEG.
“We have half a dozen patients with
varying degrees of disability who have managed to control a
robot with their thoughts,” says Professor José Millán of EPFL.
“We are also running experiments with patients controlling
wheelchairs. And we have shown that people can open and close
their own hands, by thinking about it and sending signals to an
FES device.”
At the University of Western Ontario in
Canada, Adrian Owen and colleagues are using EEG to show that some
patients with severe brain injuries, who seem to be minimally
conscious or in a vegetative state, actually display the mental
signature of full consciousness.
For example, when the researchers ask
the patients to imagine moving their right and left hand and toes,
about 20 per cent of them produce patterns of brain activity
indistinguishable from a healthy person following the same commands.
“It’s astonishing,” says Prof Owen.
“In some of these cases, patients who seemed to be entirely
unresponsive to the outside world were able to signal that they
were in fact conscious by changing their pattern of brain
activity - sometimes hundreds of times.”
Now researchers at Western Ontario and
elsewhere are working to translate these findings into a system that
would unlock the brains of people who are too paralyzed even to
communicate by blinking their eyes to order.
“We want to get to the stage where
they can spell out words with characteristic brain activity for
each letter of the alphabet - and we can work out in real time
what they are saying,” Prof Owen says.
Such a system might pose some ethical
dilemmas, like someone spelling out:
“I want to die.”
“But if a patient feels that way, it
is surely better that he or she should be able to express it,”
he says.
While enabling people who are completely
locked in to communicate - or tetraplegics to walk - might be the
most striking demonstrations of neurotechnology, treatments for less
serious disabilities and for brain diseases represent a vastly
greater market.
Epilepsy is a tempting target. It is one of the most common
neurological disorders, affecting 50m people worldwide; it is poorly
controlled with conventional drugs; and it is caused directly by
electrical malfunctioning of the brain.
Several researchers are using bioelectronics to investigate exactly
where the abnormal electrical firing starts and how it spreads
across the brain. Then, armed with this knowledge, they hope to
intervene to prevent seizures.
Some of the best bioengineers in the US, including John Rogers of
the University of Illinois, Brian Litt of the University of
Pennsylvania and Jonathan Viventi of New York University, are
developing a new generation of soft computer interfaces, made with
flexible silicon circuitry that can bend and stretch to match the
curves of the brain.
Their “smart
skin” is particularly well suited to investigate and
later treat epilepsy.
It will be implanted under the skull,
lying on top of the brain but not penetrating the grey matter.
“We are gathering safety data from
animals before going into the clinic,” says Prof Viventi. “We
hope there will be human testing within a year or two, to see
how seizures move across the brain.”
The consumer electronics industry is
developing headsets that claim to let users control computers and
games through their thoughts.
But neuroscientists say these are too
crude for clinical applications.
“My life is dogged by people saying
we should borrow from the games industry,” says Prof Owen.
“Unfortunately their devices are useless for our purposes. A lot
of them are really just picking up muscle rhythms rather than
real neural activity.”
Neuromarketing companies also use EEG
caps to measure consumer preference.
But again Prof Owen is
dismissive:
“Observing brain signals in someone
choosing between Pepsi and Coke is very different from driving a
prosthetic arm.”
Several small companies are active in
clinical bioelectronics. For example mc10, based in Massachusetts,
is commercializing the smart skin developed by Prof Rogers and
colleagues.
There are also companies working in two clinical fields that have
become established in relative isolation from the rest of
bioelectronics research: cochlear implants translate sound into
auditory signals for deaf people; “deep brain stimulation” reduces
tremor in Parkinson’s patients, though its precise mechanism of
action remains uncertain.
Commercialization remains fragmentary, however, says Prof Donoghue.
“We’re at a threshold where we need
input from industry, a partnership between clinicians and
industry.”
Although bioelectronics research
currently focuses on the brain, its potential extends much wider.
“Electrical signaling is not
confined to the central nervous system,” says Mr Slaoui. “We
could make use of signaling in the peripheral nervous system
too.”
For instance, it might be possible to
treat obesity by sending local signals to cells in the stomach or
gut that would control the patient’s appetite, without involving the
brain.
Or, if cells in the pancreas fail to
make enough insulin in diabetic patients, a signal could be sent to
cells elsewhere to do the job instead.
“The beauty of electrical signalling
is that it has only a local effect,” says Mr Slaoui. In
contrast, even a well- targeted drug drenches the whole body in
unwanted chemical.
Mr Slaoui senses that this is a moment
comparable to the birth of the modern pharmaceuticals industry at
the end of the 19th and beginning of the 20th century, when
chemicals companies began to realize that they could design
compounds with a biological effect.
If bioelectronics achieves even half as much as GSK expects, it will
transform the drugs industry over the next generation or two.
|