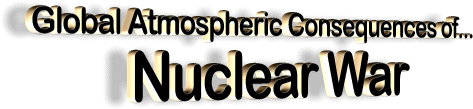
by R. P. Turco, O.B. Toon,
T.P. Ackerman, O.B. Pollack and Carl Sagan
25 July 1983
from
NASATechnicalReportsServer Website
Abstract
We investigate the global atmospheric and climatic consequences of
nuclear war for a wide range of detonation yields and scenarios, and
for variations of uncertain physical parameters over their plausible
ranges of values.
Significant hemispherical (and possibly
global) attenuation of the solar radiation flux (to less than ten
percent of ambient for several weeks) and cooling of continental
land areas (by 30 K or more for 1 to 2 months) may be caused by
smoke generated in city and forest fires ignited by airbursts of.
all yields, and by fine dust raised in high-yield surface bursts.
A limited number of nuclear explosions
around urban centers, amounting to only ~ 100 megatons of total
yield, can create smoke optical depths > 1 for weeks and, even in
summer, subfreezing land temperatures for months. In most, of the
simulated exchanges involving several thousand megatons, in which
both dust and smoke are generated, light levels are initially
reduced to a few percent of ambient, and temperature minima are <
250 K.
Radioactive fallout on intermediate
timescales (days to weeks) can lead to mean doses of ionizing
radiation (from external plus internal emitters) of 100 rads or more
at Northern mid-latitudes.
Introduction
Concern has been raised over the short - and long-terra consequences
of the dusk - smoke, radioactivity and toxic vapors generated by a
nuclear "war" (1-7). The discovery that dense clouds of
soil particles may have played a major role in past mass extinctions
of life on Earth (8-10) has encouraged our reconsideration of
nuclear war effects. It has also been suggested that massive fires
ignited by nuclear explosions could generate immense quantities of
sooty smoke, which attenuates sunlight strongly (7).
These recent developments have led us to
calculate, using new data and improved models, the climatic effects
of dust and smoke clouds (henceforth, nuclear dust and nuclear
smoke) generated in a nuclear war (11).
After a full exchange, thousands of such
clouds, produced by individual explosions and merging with one
another, could blanket northern mid-latitudes in days, altering the
atmospheric radiation balance and eventually perturbing the
circulation and climate on a global scale. Possible rapid transfer
of tropospheric and stratospheric dust and smoke from the Northern
to the Southern Hemisphere, ignored in previous investigations,
could involve the entire globe in the aftereffects.
Below, we discuss the long-term impacts of nuclear warfare, but
deliberately neglect the short-term effects of blast, fire and
radiation, which would surely represent, by themselves, an
unprecedented human catastrophe (12-14). However, most of
the world's population could survive the initial nuclear exchange,
and would inherit the post-war environment. Accordingly, the
global-scale aftereffects of nuclear war might prove to be as
important as, or more important than, the conflict itself.
To study these phenomena, we employ a series of physical models: a
nuclear scenario model, a particle microphysics model, and a
radiative-convective model. The nuclear scenario model calculates
the altitude-dependent dust, radioactivity, smoke and N0X injections
for each explosion in a nuclear exchange (given the size, number and
types of detonations, including heights of burst, geographical
locales and fission yield fractions).
The source model parameterization is
discussed below, and in a detailed report (15). The
one-dimensional microphysical model (15-17) predicts the
time evolution of horizontally-dispersed dust and smoke clouds. The
radiative-convective model utilizes the predicted dust and smoke
particle size distributions and optical constants, and Mie theory to
calculate visible and infrared optical properties, light fluxes, and
air temperatures as a function of time and height.
The techniques used in these
calculations are also well documented (15-18).
Scenarios
We have reviewed the world's nuclear arsenals (19-23). The primary
strategic and theater weapons amount to ~ 12,000 megatons (MT) of
yield carried by ~ 17,000 warheads. While the total number of
high-yield warheads is declining with time, roughly 7,000 MT may
still be accounted for by warheads > 1 MT.
These awesome arsenals munitions which
are ignored in this analysis. Scenarios for the possible use of
nuclear weapons are complex and controversial. Historically, studies
of the long-term effects of nuclear war have focused on a full-scale
exchange in the range of 5,000 to 10,000 MT (2, 12, 19).
Such exchanges are possible, given the current arsenals, and the
unpredictable nature of warfare, particularly nuclear warfare, in
which escalating massive exchanges are likely (24).
An outline of the scenarios adopted here is presented in Table 1
(below-left image).
The baseline scenario assumes a net exchange of 5,000 MT. Other
cases span a range of total yield from 100 to 25,000 MT. Many
high-priority military and industrial assets are located near or
within urban zones (25). Accordingly, a modest fraction
of the total yield is assigned to urban/industrial targets.
Owing to the large yields of strategic
warheads (> 100 kilotons, KT), "surgical" strikes against individual
targets are difficult; e.g., a 100 KT airburst can level and burn an
area of ~ 50 km2, and a 1 MT burst, ten times that area
(26, 27).
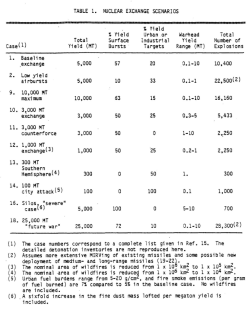 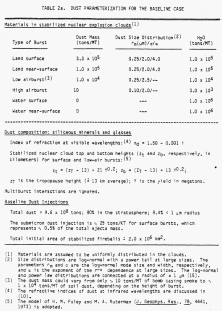 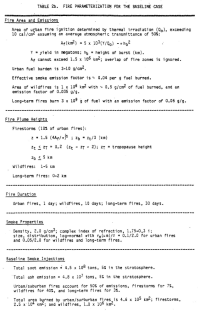
The properties of nuclear dust and smoke
are critical to the present analysis.
The basic parameterizations
are described in Tables 2a and b (above images, center and right), respectively.
For each explosion
scenario, the fundamental quantities which must be known to make
optical and climate predictions are the total atmospheric injections
of fine dust (< 10 um radius) and soot.
Nuclear explosions at or near the ground can generate fine particles
by several mechanisms (26):
-
ejection and disaggregation of
soil particles (28)
-
vaporization and re-nucleation
of earth and rock (29)
-
blow-off and sweep-up of surface
dust and smoke (30)
Analyses of nuclear test data indicate
that roughly 1-6 x 102 tons of dust per megaton of
explosive yield are held in the stabilized clouds of land surface
detonations (31). Moreover, size analysis of dust samples
collected in nuclear clouds indicates a substantial submicron
fraction (32).
The data suggest that nuclear surface
detonations may be much more efficient in generating fine dust than
volcanic eruptions (15), which have been used
inappropriately in the past to calibrate the impacts of nuclear war
(2).
The intense light emitted by a nuclear fireball is sufficient to
ignite flammable materials over a wide area (26). The
explosions over Hiroshima and Nagasaki both initiated massive
conflagrations (33). In each city, the region heavily
damaged by blast was also consumed by fire (34).
Assessments carried out over the past two decades strongly suggest
that widespread fires would occur following most nuclear bursts over
forests and cities (35-41).
The Northern Hemisphere has = 4 x 107
km2 of forest land, and holds an average = 2.2 g/cm2
of fuel (7).
The world's urban and suburban zones
cover an area = 1.5 x 106 km2, with an average
fuel burden = 2-5 g/cm2 (15); central cities,
which occupy an area = 50,000 km2, have fuel loads = 10-40
g/cm2 (39, 40, 42, 43). The smoke emissions of wildfires
and large-scale urban fires probably lie in the range ~ 2-8% by mass
of the fuel burned (44).
The highly-absorbing sooty fraction
(principally graphitic carbon) of the smoke could vary from 5-30% by
weight (45, 46). In wildfires, and probably in urban
fires. > 90% of the smoke mass consists of particles < 1 urn in
radius (47). For optical calculations, smoke particles
were assigned an imaginary part of the refractive index of 0.3
(48).
A number of calculations were made to test the sensitivity of the
results to the choices of the physical parameters. These included
the nuclear dust size distribution function, the total dust lofted,
smoke emission fractions, fire plume heights, aerosol optical
constants, particle agglomeration rates and rainout lifetimes, and
cloud dispersal rates.
The most significant findings of the
sensitivity analysis are summarized below.
Simulations
The model predictions discussed here generally represent effects
averaged over the Northern Hemisphere (N.H.). The initial nuclear
explosions and fires would be largely confined to northern
mid-latitudes (~ 30°N to 60°N) (49).
Zonal spreading following a massive
exchange might occur within a few days, especially since the dust
and smoke clouds would initially be widely distributed in longitude
(15). Accordingly, the predicted dust and smoke opacity
could be larger by a factor of 2 to 3 at mid-latitudes, but smaller
elsewhere.
The hemispherically-averaged visible
wavelength extinction optical depths (50) of the mixed
nuclear dust and smoke clouds corresponding to the scenarios listed
in Table 1 are shown in Figure 1 far below. For the purpose of discussion,
the vertical optical .depth provides a convenient diagnostic of
cloud properties, which may be used roughly to scale atmospheric
light levels and temperatures, for the various scenarios.
In the baseline scenario (Case 1, 5,000 MT), the initial optical
depth is ~ 4, of which ~ 1 is due to stratospheric dust and ~ 3 to
tropospheric smoke. After 1 month the optical depth is still ~ 2.
Beyond 2-3 months, dust dominates the optical effects, as the soot
is largely depleted by rainout and washout (52). In the baseline
model, about one-third of the world's urbanized land area, or ~
480,000 km2, is partially burned by ~ 1,000 MT of
explosions (which represents just 20% of the net exchange yield).
The mean quantity of fuel consumed over
this area is ~ 1.9 g/cm2, compared to ~ 2-5 g/cm2
in residential areas and s 10-40 g/cm2 in city centers
(15, 42). Wildfires ignited by the remaining 4,000 MT of
yield are assumed to burn another 1 x 106 km2
of forest, brush, and grasslands (7, 37), consuming s 0.5 g/cm2
of fuel in the process (7). The total smoke emission in
the baseline model is 450 million tons (released over several days);
by comparison, current global smoke emissions (released over one
year) are estimated as ~ 200 million tons (15).
The optical depth simulations for Cases 1, 2, 9 and 10 in Figure 1
far below, show that a range of exchanges between 3,000 and 10,000 MT might
cause nearly the same effects. Even Cases 11, 12 and 13, while less
severe in their absolute impact, produce optical depths comparable
to or exceeding those of a major volcanic eruption.
It is noteworthy that eruptions such as
Tambora in 1815 may have produced historically significant climate
perturbations (53-55).
Unexpectedly, Case 14, a limited 100 MT airburst attack over cities,
generates large smoke opacities over at least a hemisphere. In this
scenario, the cores of ~ 200 cities are partially burned. Most large
cities hold important strategic targets, which suggests that, in any
nuclear exchange, a substantial number of explosions would occur in
urban settings where combustible materials are concentrated.
Strategic warheads targeted on cities lie in the > 100 KT range, and
are capable of igniting fires over large areas adjacent to targets.
These facts imply a relatively low
threshold for major optical and climatic consequences in a nuclear
war.
Figure 2 gives the surface temperature perturbation over land
calculated from the dust and smoke optical depths for several
exchange scenarios. Most striking are the extremely low temperatures
occurring within 3-4 weeks after a major exchange. In the baseline
5,000 MT model, a minimum temperature of ~ 250 K (-23°C) is
predicted after 3 weeks. Subfreezing temperatures persist for
several months. Among the cases shown, even the smallest temperature
decreases are ~ 5-10°C (Cases 4, 11 and 12).
Since average surface temperature
changes of only 1°C are considered significant (56),
severe climatological consequences might be expected in each of
these cases. Even the 100 MT city airburst scenario (Case 14)
produces a two-month interval of subfreezing land temperatures, with
a minimum again near 250 K. The temperature recovery in this
instance is hastened by the absorption of sunlight in optically thin
late soot clouds (see below).
Comparable exchanges with and without
smoke emission (e.g., Cases 10 and 11) show that the tropospheric
soot layers cause a sudden surface cooling of short duration, while
fine stratospheric dust is responsible for prolonged cooling lasting
a year or more.
In all cases considered, nuclear dust acts to cool the Earth's
surface. Soot also tends to cool the surface except when the soot
cloud is both optically thin and located near the surface [an
unimportant case because only relatively small transient warmings <
2K can thereby be achieved (57)].
The predicted temperature variations of the world's oceans due to
radiative transport alone are always small (~ 3 K cooling), owing to
the great heat capacity and rapid mixing of surface waters. However,
changes in the atmospheric zonal circulation patterns (see below),
might significantly alter ocean currents and upwelling, as has
occurred on a smaller scale recently in the Eastern Pacific ("El
Niño").
The oceanic heat reservoir would also
moderate the predicted continental land temperature decreases,
particularly in coastal regions (10). The effect on
interior continental temperatures is difficult to assess due to
possible changes in atmospheric circulation patterns.
The predicted temperature changes (from ambient values) for the
baseline nuclear exchange are illustrated as a function of time and
altitude in Figure 3. The dominant features of the temperature
perturbation are a large warming (up to 80 K) occurring in the lower
stratosphere and upper troposphere and a large cooling (up to 40 K)
of the surface and lower troposphere. The warming is caused by the
absorption of solar radiation in the upper-level dust and smoke
clouds, and persists for an extended period due to the long
residence time of the particles at great elevations.
The strength of the warming is due both
to the low thermal inertia of the atmosphere (because of its low
density) and the initially cold temperatures at high altitudes. The
low-level cooling is the result of the attenuation of the incident
solar radiation by the aerosol clouds (see Figure 4) during the
early period of the simulation. No greenhouse effect occurs, because
the solar energy is deposited above the height at which infrared
energy is radiated to space.
The present results do not reflect the strong coupling between the
modified heating/cooling rates computed here and the bulk
atmospheric motions. Global circulation patterns would probably be
altered in response to such large disturbances in the driving
forces.
However, the prediction of circulation anomalies is beyond
the capability of our 1-d radiative-convective model.
Decreases in insolation for several nuclear war scenarios are given
in Figure 4. The baseline case implies average solar fluxes at the
ground < 10% of normal values for several weeks. In addition to
causing the temperature declines discussed above, the attenuated
insolation could affect plant growth rates, and vigor in the marine
(58), littoral and terrestrial food chains.
In the 10,000 MT "severe" case, average
light levels are below the minimum required for photosynthesis for
about 40 days over much of the N.H. In a number of other cases,
insolation may, for more than two months, fall below the
compensation point,, at which photosynthesis is insufficient to
maintain plant metabolism.
Because the nuclear clouds are likely to
remain patchy the first few weeks after an exchange, the leakage of
sunlight through holes in the clouds could enhance the levels of
plant growth activity above those predicted for average cloud
conditions.
Sensitivity
Tests
Figure 5 shows the effects of variations in the nuclear dust
parameters on the baseline dust cloud opacity (with soot excluded).
The initial dust optical depths vary from ~ 0.2 to ~ 3.
Nuclear dust alone could produce a
significant climatological excursion, particularly if the submicron
component is as much as 20-50 tons/KT. However, if the submicron
particles comprise < 10 tons/KT, the dust optical effects would be
no worse than for a major volcanic eruption. In the baseline case,
the dust opacity is much greater than the opacity associated with
the
El Chichon and
Agung eruptions (18, 59).
Figure 5 compares cloud optical depths for several variations of the
baseline model smoke parameters (with dust included). In the
baseline case, it is assumed that firestorms inject only a small
fraction (~ 5%) of the total smoke emission into the stratosphere
(60). Thus, Case 1 and Case 3 (no firestorms) are very
similar. However, if all the smoke is injected into the stratosphere
and rapidly dispersed around the globe, Case 26 shows that large
optical depths might endure for a year (Figure 6).
A prolongation of optical effects is
also obtained in Case 22, where the tropospheric washout lifetime of
smoke particles is increased from 10 to 30 days near the ground. By
contrast, when the nuclear smoke is initially contained near the
ground, and the dynamical and hydrological processes are assumed to
be unperturbed, smoke removal is much faster (Case 25). Some of the
smoke still diffuses to the upper troposphere and remains there for
several months (61).
If the smoke is composed mainly of oily compounds, the particle
density may be closer to 1 g/cm2 than to the baseline
value of 2 g/cm2 (Ref. 48). Case 28 in Figure 6 (far
below) shows
that the smoke opacity is roughly doubled when the lower density is
used (i.e., for a fixed smoke mass emission and size distribution,
the particle number density and optical depth vary inversely with
the bulk density).
On the other hand, the optical depths
calculated for smoke imaginary refractive indices between 0.1 and
0.3 show virtually no differences (Cases 1 and 27). In other
calculations, the imaginary refractive index was allowed to vary
between 0.3 and 0.01. At a value of 0.05, the absorption optical
depth (50) was reduced by only ~ 50%, and at a value of
0.01, by ~ 85%.
The overall extinction, moreover,
increased by ~ 5%. These results demonstrate that light absorption
and heating rates in the nuclear smoke clouds are not very sensitive
to graphitic carbon fractions larger than a few percent.
One sensitivity test (Case 29, not illustrated) considers the
optical effects in the Southern Hemisphere (S.H.) of
soot and dust transported from the N.H. stratosphere. In this
calculation, the smoke in the 300 MT S.H. Case 13 is combined with
half the baseline stratospheric dust and smoke (to approximate rapid
global dispersion in the stratosphere).
The initial optical depth is ~ 1 over
the S.H., dropping to about 0.3 in 3 months. The predicted average
S.H. continental surface temperatures fall by 8 K within several
weeks and remain at least 4 K below normal for nearly 8 months. The
seasonal influence should be taken into account, however.
For example, the worst consequences for
the N.H. might result from a spring or summer exchange, when crops
are vulnerable and fire hazards are greatest. The S.H., in its fall
or winter, might then be least sensitive to cooling and darkening.
Nevertheless, the implications of this scenario for the tropical
regions in both hemispheres would appear to be serious, and worthy
of further analysis. Seasonal factors can also modulate the
atmospheric response to perturbations by smoke and dust, and should
be considered.
A number of sensitivity tests for more "severe" cases were run with
scenario yields ranging from 1,000 to 10,000 MT and smoke and dust
parameters assigned larger, but not implausible, values. The
predicted effects are clearly substantially worse. The lower
probabilities of these assigned parameter values must be weighed
against the catastrophic outcomes which they imply.
Prudent policy would calibrate the
importance of these scenarios as the product of their probabilities
and the costs of their corresponding effects. Unfortunately, we are
unable to give an accurate quantitative estimate of the relevant
probabilities. By their very nature, however, the severe cases may
be the most important to consider in the deployment and use of
nuclear weapons.
With these reservations in mind, we present the optical depths for
some of the more "severe" cases in Figure 7. Large opacities can
persist for a year, and land surface temperatures can fall to
230-240 K, or about 50 K below normal. Combined with low light
levels (Figure 4 - far below), these scenarios raise the possibility of
catastrophic widespread ecological consequences.
Two sensitivity tests were run to determine roughly the implications
for optical properties of aerosol agglomeration in the early
expanding clouds (the simulations already take into account the
continuous coagulation of the particles in the dispersed clouds).
Very slow dispersion of the initial stabilized dust and smoke clouds
over the N.H., taking nearly 8 months, was assumed. Coagulation of
particles reduced the average opacity after 3 months by about 40%.
When the adhesion efficiency of the colliding particles was also
maximized, the average opacity after 3 months was reduced by ~ 75%.
In the most likely situation, however,
prompt agglomeration and coagulation might reduce the average
hemispherical cloud optical depths by 20-50%.
Other Effects
We have also considered, in a less rigorous manner, the long-term
effects of radioactive fallout, fireba11-generated NOX, and pyrogenic toxic gases (15). The physics of radioactive
fallout is well known (2,5,12,26,62).
The present calculations bear primarily
on the widespread accumulation of intermediate-timescale fallout due
to dry deposition and washout of dispersed nuclear dust (63).
Predicted average chronic whole-body gamma ray doses for the
baseline scenario (5000 MT) are illustrated in Figure 8.
For exposure only to the radioactive
dust which begins to fall out after a few days, the
hemisphere-average total dose accumulated over several months would
be 20 rad. Fallout during this time would be confined largely to
northern mid-latitudes; hence the dose there could be ~ 2-3 times as
large (64). Considering the accompanying ingestion of
biologically active radionuclides (26, 65) and occasional
exposure to localized fallout, we estimate an average chronic
mid-latitude dose of ionizing radiation for the baseline case > 100
rad (66).
In a 10,000 MT exchange, this mean dose
would be doubled.
The stratospheric nuclear-NOX/ozone problem has been treated in a
number of studies (2-4, 7, 67). The present calculations,
using updated chemical rate coefficients, give roughly the same
results as these earlier investigations (15).
Accordingly, detailed results are not reviewed here. In Figure 9
(far below),
however, nuclear-NOx injection profiles are shown for
several war scenarios.
Owing to the rather direct dependence of
ozone depletion on stratospheric NOx injection, a high
sensitivity of the ozone perturbation to the scenario may be
inferred. In the baseline case, a maximum hemispherically-averaged
ozone reduction of ~ 30% is found. However, this would be.
substantially smaller is most yields of individual warheads were < 1
MT.
Considering the relationship between
solar UV-B radiation increases and ozone decreases (68),
UV-B doses roughly twice normal are expected in the first year
following a baseline exchange (after the dust and soot had
dissipated). Larger UV-B effects could accompany exchanges involving
warheads of larger yields (or large multi-burst lay-downs).
A variety of toxic gases would be generated in large quantities by
nuclear fires, including CO and HCN.
According to Crutzen and Birks (7),
heavy air pollution, including elevated ozone concentrations, could
blanket the N.H. for several months. We are also concerned about
dioxins and furans, extremely persistent and toxic compounds
released during the combustion of widely-used synthetic organic
chemicals (69). Hundreds of tons of dioxins and furans
could be generated during a nuclear exchange (70).
The long-term ecological consequences of
these compounds also seems worthy of future consideration.
Meteorological Perturbations
Thick clouds of nuclear dust and smoke can cause significant
climatic perturbations, and related effects, through a variety of
mechanisms:
-
reflection of solar radiation to
space leading to an overall surface cooling
-
modification of solar absorption
and heating patterns which drive the atmospheric
circulation-on small scales (71) and large scales
(72)
-
introduction of numerous cloud
condensation nuclei which affect the formation of clouds and
precipitation (73)
-
alteration of the surface albedo
by fires and soot (74)
These effects are closely coupled in
determining the overall response of the atmosphere to nuclear war
(75).
It seems very difficult at present to
forecast in any detail the changes in coupled atmospheric
circulation and radiation fields, and in weather and microclimates,
for the massive dust and smoke injections treated here. Hence,
speculation must be limited to the most general considerations.
Water evaporation from the oceans implies a continuing source of
moisture for the marine boundary layer. A heavy semi-permanent fog
or haze layer might blanket large bodies of water. The consequences
for marine precipitation are unclear, particularly if the normal
prevailing winds are greatly modified by the perturbed solar driving
force.
Cold air from the continents might
circulate as offshore winds in coastal regions, causing heavy
precipitation in these areas, and inducing a return flow aloft,
possibly containing substantial amounts of condensed water. Certain
continental zones might be subject to continuous snowfall for
several months (10). Precipitation can lead to soot
removal, although this process may not be very efficient for nuclear
clouds (71, 73).
It is likely that, on average,
precipitation rates would be generally smaller than in the ambient
atmosphere; the major remaining energy source available for storm
genesis is the latent heat from ocean evaporation.
Despite possible heavy snowfalls, it is improbable that an Ice Age
would be triggered by a nuclear war. The period of cooling (~ 1
year) is in all likelihood too short to overcome the considerable
inertia in the Earth's climate system. The oceanic heat reservoir
would probably force the climate toward contemporary norms in the
years following a war.
The CO2 input from nuclear
fires is not significant climatologically (7).
Inter-hemispheric Transport
Earlier studies assumed that significant inter-hemispheric transfer
of nuclear debris and radioactivity requires a year or more (2).
This perception was based on observations of transport under ambient
conditions, including the dispersion of debris clouds from
atmospheric nuclear weapons tests. However, with dense clouds of
dust and smoke, as described above, massive dynamical disturbances
would be expected in the aftermath of a nuclear war.
A rough analogy can be drawn with the
evolution of global-scale dust storms on Mars. The lower Martian
atmosphere is similar in density to the Earth's stratosphere, and
the period of rotation is almost identical to the Earth's. Dust
storms that develop in one hemisphere on Mars often rapidly
intensify and spread over the entire planet, crossing the equator in
a mean time 5 10 days (15, 76, 77).
The explanation apparently lies in the
heating of the dust aloft, which then dominates other heat sources
and drives the circulation. Haberle et al. (76) simulated
the evolution of Martian dust storms and found that dust at low
latitudes, in the core of the Hadley circulation, is the most
critical in modifying the winds. In a nuclear exchange, most of the
dust and smoke would be injected at-middle latitudes.
However, Haberle et al. (76)
did not include planetary-scale waves in their calculations.
Perturbations of planetary wave amplitudes may be critical in the
transport of nuclear war debris between middle and low latitudes.
There appear to be four distinct mechanisms which could produce
significant atmospheric effects in the S.H.:
-
Direct soot injection following
targeting of S.H. cities by as few as 100 MT (e.g., Case 14,
Figures 1, 2 and 4 - far below).
-
Normal tropospheric wind
patterns. Because the "meteorological equator" lies near 5°N
latitude, some of the debris from nuclear explosions below
20-30°N (e.g., in India and South China) could be carried
into the S.H., particularly in the monsoon season (78).
-
Normal inter-hemispheric
exchange of stratospheric air. For example, photometric
observations of the ET Chichon volcanic cloud (origin, 14°N)
by the Solar Mesospheric Explorer satellite show that
perhaps 10-20% of the aerosol material had been transported
to the S.H. after ~ 7 weeks (79).
-
Unprecedented tropospheric and
stratospheric transport driven by temperature gradients
between the hot, dust - and soot-laden N.H. upper atmosphere
and the cold, initially-clear, S.H. upper atmosphere.
Resolution of the problem of accelerated
inter-hemispheric transport following a large nuclear exchange must
await, at the least, more detailed general circulation model
calculations.
The response of the atmosphere could
turn out to be sensitive to the quantities, altitudes, and
geographical locations of the dust and smoke injections, which are
uncertain. The implications of rapid inter-hemispheric transport are
serious.
If global-scale mixing can occur within
a few weeks, the S.H. could be subjected to severe environmental
stresses even in the absence of a direct attack.
Conclusions
The studies outlined here suggest severe long-term climatic effects
from a paroxysmal 5000 MT nuclear exchange.
Despite uncertainties in
the amounts and properties of the dust and smoke produced by nuclear
detonations, the following tentative conclusions may be drawn:
-
Contrary to the findings of
earlier studies [e.g., (2)], global nuclear war might have a
major impact on climate — manifested by an unbroken overcast
for many weeks, subfreezing land temperatures persisting for
several months, large perturbations in global circulation
patterns, and dramatic changes in local weather and
precipitation rates — a harsh "nuclear winter" in any
season.
Greatly accelerated
inter-hemispheric transport in the stratosphere might also
occur, although modeling studies are needed to quantify the
importance of this effect; Rapid inter-hemispheric mixing
implies that the Southern Hemisphere could be subjected to
massive injections of nuclear debris soon after an exchange
in the Northern Hemisphere. In apparently all previous
studies, S.H. effects have been assumed to be minor.
Although the climate
disturbances are expected to last for several years, it
seems unlikely (although still uncertain) that a major
long-term climatic change, such as an Ice Age, would be
triggered.
-
Relatively large climatic
effects might result even from small nuclear exchanges (~
100 to 1000 MT), because fires can be initiated by nuclear
weapons of any size, and as little as ~ 100 MT is sufficient
to devastate most of the world's major urban centers. The
existence of such a low threshold yield for massive smoke
emissions implies that even limited nuclear exchanges could
trigger severe aftereffects. It is much less likely that a
5,000-10,000 MT exchange would produce only relative minor
effects.
-
The climatic impact of sooty
smoke from nuclear fires' ignited by airbursts is probably
more important than dust raised by surface bursts (when both
effects occur). Smoke is generally highly-absorbing, whereas
soil dust is typically non-absorbing. Smoke particles are
extremely small, which lengthens their atmospheric residence
times. There is also a high probability that nuclear
explosions over cities and forests will initiate widespread
fires.
-
Smoke from urban fires may be
more important than smoke from collateral forest fires for
two reasons:
-
in a full-scale exchange,
cities holding large stores of combustible materials
would be attacked directly
-
intense firestorms could
pump smoke into the upper atmosphere, where the
residence time is a year or more.
-
Nuclear dust can also contribute
to the climatic impact of a nuclear exchange. Previous
analogies with volcanic eruptions have been misinterpreted.
The dust-climate effect is very sensitive to the conduct of
the war; a smaller effect is expected when lower yield
weapons are deployed and air bursts dominate over surface
land bursts. Multiburst phenomena might synergistically
enhance nuclear dust climatic effects, but insufficient data
are available to assess this problem.
-
Exposure to radioactive fallout
may be more intense and widespread than is predicted by
standard empirical exposure models, . which neglect the
intermediate fallout extending over many days and weeks,
particularly when unprecedented quantities of fission debris
are released abruptly in the atmosphere. Average
mid-latitude whole-body gamma ray doses of 50-100 rad are
possible in a 5000 MT exchange, with much larger doses
within the fallout plumes of radioactive debris extending
hundreds of kilometers downwind of targets.
-
Synergisms between long-term
nuclear war stresses - e.g., low light levels, subfreezing
temperatures, exposure to intermediate timescale radioactive
fallout, heavy air pollution, and UV-B flux enhancements -
aggravated by the destruction of medical facilities, food
stores, and civil services, could lead to many additional
fatalities, and could place the global ecosystem in serious
jeopardy.
Our estimates of nuclear war effects are
uncertain because the problem is too complex to resolve precisely
with existing analytical tools, because the data base is incomplete,
and because the problem is not amenable to experimental
investigation.
In particular, we are unable to forecast
the detailed nature of the changes in atmospheric dynamics and
meteorology implied by our nuclear war scenarios, nor the effect of
such changes on the maintenance or dispersal of the initiating smoke
and dust clouds.
Nevertheless, the magnitudes of the
first-order effects are so large, and the implications so serious,
that we hope the scientific issues raised here will be vigorously
and critically examined (79).
FIGURE CAPTIONS
-
Time-dependent hemispherically-averaged
vertical optical depths (extinction = scattering plus
absorption) of nuclear smoke and dust clouds at 550 nm
wavelength. Optical depths < -0.1 are negligible, ~ 1 are
significant, and ~ 10 imply possible major consequences. The
transmission of sunlight becomes highly nonlinear at optical
depths > 1. Results are given for several of the scenarios
listed in Table 1 (image far above.)
Calculated optical depths for the
expanding El Chichon eruption cloud are offered for
comparison (51).
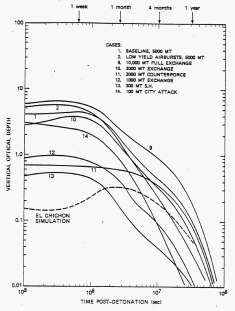
-
Hemispherically-averaged surface
temperature variations following a nuclear exchange. Results
are shown for several of the scenarios in Table 1. (Note the
linear timescale, unlike that in Figure 1). The temperatures
generally apply to the interior of continental land masses.
In Cases 4 and 11, fires are neglected. In the other cases,
smoke contributes to the temperature effect in varying
degrees.
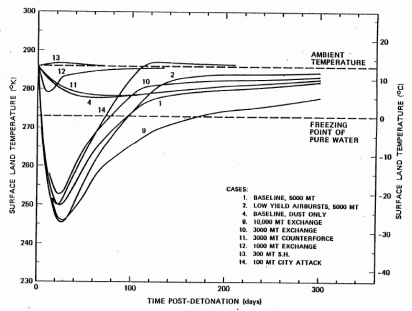
-
Northern Hemisphere troposphere
and stratosphere temperature perturbations in K° (or in C°)
following the baseline nuclear exchange (Case 1). The
cross-hatched area indicates cooling.
Pressures are given in millibars.
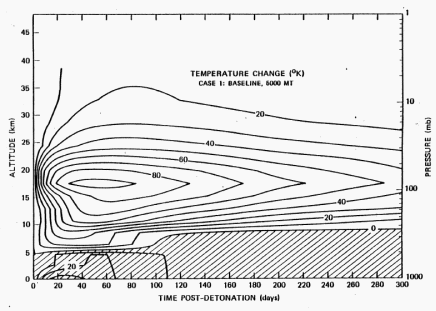
-
Solar energy fluxes at the
ground over the Northern Hemisphere in the aftermath of a
nuclear exchange. Results are given for several of the
scenarios in Table 1. (Note the linear timescale.)
The solar
fluxes are averaged over the diurnal cycle and over the
hemisphere. Cases 4 and 16 neglect fires. Also indicated on
the figure are the approximate flux levels at which
photosynthesis cannot keep pace with plant respiration
(compensation point), and at which photosynthesis ceases.
These limits vary for different species.
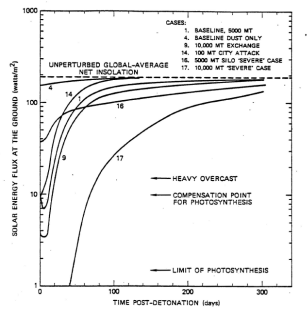
-
Time-dependent vertical optical
depths (absorption plus scattering at 550 nm) of nuclear
dust clouds, in a sensitivity analysis. The optical depths
are average values for the Northern Hemisphere.
The cases shown are:
-
4, baseline dust only, 3.3 x
105 tons/MT for surface bursts, size
distribution as described in (32)
-
5, high dust loading, 1 x 106
tons/MT
-
6, low dust loading, 1 x 105
tons/MT
-
7, coarse dust, mode radius
~ 1.0 µm
-
8, fine dust, mode radius ~
1.0 µm
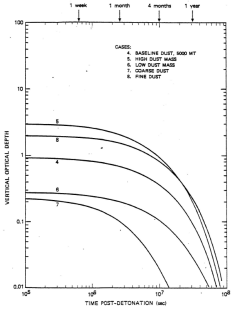
-
Time-dependent vertical optical
depths (absorption plus scattering at 550 nm) of nuclear
smoke clouds, in a sensitivity analysis. The optical depths
are average values for the Northern Hemisphere. All cases
shown correspond to parameter variations of the baseline
model and all include dust appropriate to that case (Case
1):
-
3, no firestorms
-
4 no fires
-
22, smoke rainout rate
decreased by a factor of 3
-
25, smoke initially confined
to the lowest 3 km of the atmosphere
-
26, smoke initially
distributed between 13 and 19 km over the entire globe
-
27, smoke imaginary part of
refractive index reduced from 0.3 to 0.1
-
28, smoke material density
reduced from 2 g/cm3 to 1 g/cm3
In Case 4, only dust from the
baseline case is considered (fires are ignored) for
comparison.
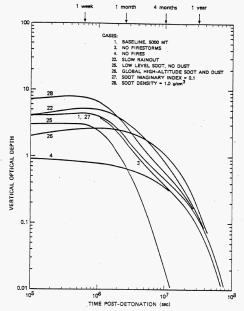
-
Time-dependent vertical optical
depths (absorption plus scattering at 550 nm) for more
severe cases of explosion yield for nuclear dust and smoke
production. The conditions are detailed in (15),
The yield inventories are identical to the nominal cases of
the same total yield described in Table 1 (Cases 16 and 18
are listed there).
The "severe" cases generally
include a sixfold increase in the fine dust injection, and a
doubling of the smoke emission. In Cases 15, 17 and 18,
smoke causes most of the opacity during the first 1-2
months. In Cases 17 and 18, dust makes a major contribution
to the optical effects beyond 1-2 months.
In Case 16, fires are neglected
and dust from surface bursts produces all of the opacity.
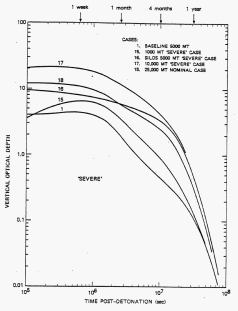
-
Hemispherically-averaged nuclear
radiation doses associated with fallout in the baseline
model (Case 1). The curves correspond to the whole-body
gamma ray doses received after a specific initial time
(abscissa) in a region where fallout began at a specific
earlier time (individual curves).
The dashed line defines the
maximum dose possible as a function of the initial fallout
time. For each curve, the exposure to fallout on the ground
is assumed to continue indefinitely.
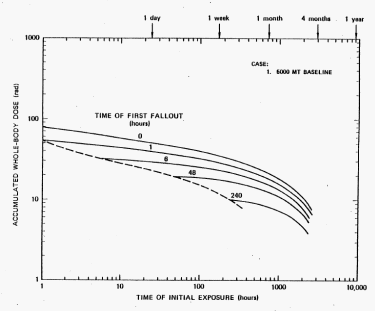
-
Nuclear NOX
injections for several exchange scenarios. The injections
are given as tons of NO per 2-km altitude interval. As a
very rough conversion, each 1 x 106 tons of
stratospheric NO injection increases UV-8 radiation
exposures at the ground by 2-4% (68).
Thus, the
baseline case corresponds to a 40 to 80% increment in UV-B
fluxes, and Case 18 corresponds to an increment by factors
of 2 to 4.
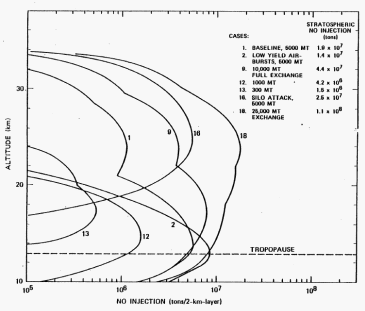
References and Notes
1. Hampson, J., Nature. 250, 189
(1974).
2. National Academy of Sciences, Long-term Worldwide Effects of
Multiple Nuclear-Weapon Detonations. 213 pp., Washington, D.C.,
1975.
3. Whitten, R. C, W. J. Borucki and R. P. Turco, Nature, 257, 38
(1975).
4. MacCracken, M. C, and J. S. Chang (Eds.), Report UCRL-51653,
Lawrence Livermore Laboratory, Livermore, CA, 77 pp., April
(1975).
5. Mark, J. C, Ann. Rev. Nucl. Sci., 26, 51 (1976).
6. Lewis, K. N., Sci. Amer., 241, 35 (1979).
7. Crutzen, P. J., and J. W. Birks, Ambio, 11, 114 (1982).
8. Alvarez, L. W., W. Alvarez, F. Asaro and H. V. Michel,
Science, 208, 1095 (1980); Alvarez, W., F. Asaro, H. V. Michel
and L. W. Alvarez, Science, 216, 886 (1982); Alvarez, W., L. W.
Alvarez, F. Asaro and H. V. Michel, Geological Soc. Amer.
Special Paper 190, L. T. Silver and P. H. Schultz, Eds., p. 305
(1982).
9. Ganapathy, R., Science, 216, 885 (1982).
10. Toon, 0. B., J. B. Pollack, T. P. Ackerman, R. P. Turco, C.
P. McKay and M. S. Liu, Geological Soc. Amer. Special Paper 190,
L. T. Silver and P. H. Schultz, Eds., p. 187 (1982); Pollack,.
J. B., 0. B. Toon, T. P. Ackerman, C. P. McKay and R. P. Turco,
Science, 219, 287 (1983).
11. The National Academy of Sciences has also undertaken a full
reassessment of the possible climatic effects of nuclear war
under the sponsorship of the Defense Nuclear Agency. The present
analysis was stimulated, in part, by a preliminary request by
the NAS to study the climatic effects of nuclear dust.
12. OTA, The Effects of Nuclear Mar, Office of Technology
Assessment, OTA-NS-89, 151 pp. (1979).
13. Coggle, 0. E., and P. J. Lindop, Ambio, 11, 106 (1982).
14. Bergstrom, S., et al.. "Effects of Nuclear War on Health and
Health Services," World Health Org. Publ. A36.12 (1983).
15. Turco, R. P., 0. B. Toon, T. P. Ackerman, J. B. Pollack and
C. Sagan, "Long-term atmospheric and climatic consequences of
nuclear war," to be submitted to J. Geophys. Res. (1983).
16. Turco, R. P., P. Hamill, 0. B. Toon, R. C. Whitten and C. S.
Kiang, J. Atmos. Sci., 36, 699 (1979); Turco, R. P., P. Hamill,
0. B. Toon, R. C. Whitten and C. S. Kiang, NASA Tech. Paper
1362, 94 pp. (1979); Turco, R. P., 0. B. Toon, P. Hamill and R.
C. Whitten, J. Geophys. Res., 86, 1113 (1981); Turco, R. P., 0.
B. Toon and R. C. Whitten, Rev. Geophys. Space Phys., 20, 233
(1982); Turco, R. P., 0. B. Toon, R. C. Whitten, P. Hamill and
R. G. Keesee, J. Geophys. Res., 88, 5299 (1983).
17. Toon, 0. B., R. P. Turco, P. Hamill, C. S. Kiang and R. C.
Whitten, J. Atmos. Sci., 36,,718 (1979); Toon, 0. B., R. P.
Turco, P. Hamill, C. S, Kiang and R. C. Whitten, NASA Tech.
Paper 1363, 67 pp. (1979).
18. Pollack, J. B., 0. B. Toon, C. Sagan, A. Summers, B. Baldwin
and W. VanCamp, J. Geophys. Res., 81, 1071 (1976); Toon, 0. B.,
and T. P. Ackerman, Appl. Optics. 20, 3657 (1981); Ackerman, T.
P., and 0. B. Toon, Appl. Optics. 20, 3661 (1981).
19. Advisors, Ambio, 11, 94 (1982).
20. Jane's Weapon Systems, 1981-1982, R. T. Pretty, Ed., Jane's
Publ. Co., London (1981).
21. The Military Balance 1982-1983, The International Institute
for Strategic Studies, 23 Tavistock Street, London (1983).
22. World Armaments and Disarmament, Stockholm International
Peace Reserch Institute Yearbook 1982, Taylor and Francis Ltd.,
London (1982).
23. Forsberg, R., Sci. Amer., 247, 52 (1982).
24. The unprecedented difficulties involved in controlling and
limiting a nuclear exchange are discussed by P. Bracken and M.
Shubik (Technology in Society, f_, 155, 1982), and by D. Ball
(Adelphi Paper 169, International Institute for Strategic
Studies, London, 1981).
25. Kemp, G., Adelphi Paper 106, International Institute for
Strategic Studies, London (1974).
26. Glasstone, S., and P. J. Dolan (Eds.), The Effects of
Nuclear Weapons, U.S. Department of Defense, 653 pp. (1977).
27. The areas quoted are subjected to peak overpressures ~ 2-3
psi and thermal irradiances > 10-20 cal/cm2.
28. A 1 MT surface explosion will eject ~ 5 x 106
tons of debris, forming a large crater (Ref. 26). Typical soils
consist of » 5-25% by weight of grains < 1 pm radius (D1Almeida,
G. A., and L. Schu'tz, J. Climate Appl. Meteorol., 22, 233,
1983; G. Rawson, private communication, 1983). The extent of
disaggregation into parent grain sizes is probably < 10%,
however (Pinnick, R. G., G. Fernandez and B. D. Hinds, Appl.
Optics, 22, 95 1983), and would depend in part on soil moisture
and compaction.
29. A 1 MT surface explosion can
vaporize 5 2-4 x 104 tons of soil material (Ref. 26), which is
ingested by the fireball. Some of the silicates and other
refractory materials later renucleate into fine glassy spheres
(Nathans, M. W., R. Thews, and I.J. Russell, in Radionuclides in
the Environment, Adv. Chem. Ser., 93, 360, 1970).
30. A 1 MT surface explosion can raise significant quantities of
dust over an area of > 100 km2 by popcorning, due to thermal
radiation, and by saltation, due to pressure winds and
turbulence (Ref. 26). Much of the dust is sucked up in the
afterwinds established by the rising fireball. Size sorting
should favor the greatest lifting for the finest particles. The
quantity of dust lofted would be sensitive to soil type,
moisture and compactness, vegetative cover, and terrain.
Probably > 1 x 10^ tons of dust can be incorporated into the
stabilized clouds in this manner.
31. Gutmacher, R. G., G. H. Higgins and H. A. Tewes, Lawrence
Livermore Laboratory Report UCRL-14397 (1983); J. Carpenter,
private communication (1983).
32. Nathans, M. W., R. Thews and I. J. Russell, in Radionuclides
in the Environment, Adv. Chem. Series, 93, 360 (1970). These
data suggest size distributions that are log-normal at small
sizes (< 3 µm) and power law (r
-α) at larger sizes. Considering
data from a number of nuclear tests, we have adopted an average
log-normal mode radius of 0.25
µm, a
α 2.0, and an exponent,
a α 4 (Ref. 15). If all particles in the stabilized clouds lie
in the range 0.01 to 1000 m radius, the adopted size
distribution has = 8% of the total mass in particles < 1
µm
radius; this fraction of the stabilized cloud mass represents <
0.5% of the total ejecta and sweep-up mass of a surface
explosion, and amounts to = 25 tons/KT of yield.
33. Ishikawa, E., and D. L. Swain (Trans!.), Hiroshima and
Nagasaki: The Physical, Medical and Social Effects of the Atomic
Bombings, Basic Books, New York, 706 pp. (1981).
34. At Hiroshima, a 5 13 KT device created a fire over 5 13 km2.
At Nagasaki, where irregular terrain inhibited mass fire
formation, a = 22 KT weapon caused a fire over = 7 km2. These
two cases suggest that low yield (< 1 MT) nuclear explosions can
readily ignite fires over an area of 5 0.3-1.0 km2/KT. This is
roughly the area contained within the « 10 cal/cm2 and the 2 psi-overpressure
contours (Ref. 26).
35. Broido, A., Bull. Atomic Sci.. 16, 409 (1960).
36. Miller, C. F., "Preliminary evaluation of fire hazards from
nuclear detonations," Stanford Research Institute Memorandum
Report, Project IMU-4021-302, 63 pp. (1962).
37. Ayers, R. U-., Environmental Effects of Nuclear Weapons,
Vol. I, Hudson Institute Report, HI-518-RR, New York (1965).
38. Martin, S. B., "The role of fire in nuclear warfare," URS
Research Co. Report URS-764 (DNA 2692F), 166 pp. (1974).
39. DCPA Attack Environment Manual, Chapter 3, U.S. Department
of Defense, Washington, D.C. (1973).
40. FEMA Attack Environment Manual, Chapter 3, "What the Planner
Needs to Know About Fire Ignition and Spread," Federal Emergency
Management Agency, CPG 2-1A3 (1982).
41. Brode, H. L.» Large-Scale Urban Fires, Pacific Sierra
Research Corp. Note 348, Santa Monica, CA (1980).
42. Larson, D. A., and R. D. Small, Analysis of the Large Urban
Fire Environment, Pacific Sierra Research Corp. Rpt. 1210, Santa
Monica, CA (1982).
43. The urban and suburban areas of cities with populations
exceeding 100,000 (about 2300 worldwide) are surveyed in (15).
Also discussed are global fuel reserves, which are shown to be
roughly consistent with known rates of production and
accumulation of combustible materials.
44. Smoke emission data for forest fires are reviewed by D. V.
Sandberg, J. M. Pierovich, D. G. Fox and E. W. Ross, Effects of
Fire on Air, Forest Service Tech. Rpt. W0-9 (1979). The largest
emission factors occur in intense large-scale fires where
smouldering and flaming conditions exist simultaneously, and the
oxygen supply may be limited over part of the burning zone.
Smoke emissions from urban fuels consisting of synthetic organic
compounds would be larger than those from forest fuels (e.g.,
C. P. Bankston, B. T. Zinn, R. F. Browner and E. A. Powell,
Comb. Flame, 41_, 273, 1981).
45. Sooty smoke is a complex mixture of oils, tars and graphitic
(or elemental) carbon. Measured benzene-soluble mass fractions
of wildfire smokes fall in the range 3 40-75% (Sandberg et al.,
Ref. 44). Most of the residue is likely to be brown to black
(the color of smoke ranges from white, when large amounts of
water vapor are present, to yellow or brown, when oils
predominate, to gray or black, when elemental carbon is a major
component).
46. Tewarson, A., in Flame Retardant Polymeric Material, Vol. 3,
M. Lewin, S. M. Atlas and E. M. Pierce, Eds., Plenum, NY, pp.
97-153 (1982). In small laboratory burns of a variety of
polymeric compounds, the emissions of "solid" materials (which
remained on collection filters after baking at 100°C for 24
hours) ranged from 5 1-15% by weight of the carbon consumed; of
low volatility liquids, - 2 to 35%, and of high volatility
liquids, * 1 to 40%. The optical extinction of the
smokegenerated by a large number of samples varied from ~
0.1-1.5 m2 per gram of fuel burned.
47. In wildfires, the particle number mode radius is typically ~ 0.05 um (Sandberg et al., see Ref. 44). For burning synthetics,
the number mode radius can be substantially greater, but a
reasonable average value is ~ 0.1 um (e.g., C. P. Bankston, B.
T. Zinn, R. F. Browner and E. A. Powell, Comb. Flame, 41, 273,
1981). Often, larger debris particles and firebrands are swept
up by powerful fire winds, but these have short atmospheric
residence times and are not included in the present estimates
(C. K. McMahon and P. W. Ryan, Paper 76-2.3, 69th Annual
Meeting, Air Pollution Control Assoc., Portland, Oregon, June
27-July 1, 1976). Nevertheless, because winds exceeding 100
km/hr may be generated in large-scale fires, significant
quantities of fine noncombustible surface dust and explosion
debris (e.g., pulverized plaster) might be lifted in addition to
the smoke particles.
48. This assumes an average graphitic carbon fraction of about
15-30% (J. T. Twitty and J. A. Weinman, J. Appl. Meteorol., 10,
725, 1971; S. Chippett and W. A. Gray, Comb. Flame, 31, 149,
1978). The real part of the refractive index of pure'carbon is
1.75, and of many oils, is 1.5. Smoke particles were assigned a
density of 2 g/cm2; graphite has a density =2.5 g/cm2, and most
oils, < 1 g/cm2.
49. A number of targets with military, economic or political
significance can also be identified in tropical northern
latitudes and in the Southern Hemisphere (e.g., Ref. 19).
50. The direct attentuation (neglecting subsequent scattering)
of sunlight by dust and smoke particles obeys the law, I/I0 =
exp (-τ), where T is the extinction optical depth due to photon
scattering and absorption by the particles, and arccos
µ0 in the
solar zenith angle. The optical depth depends on the wavelength
of the light and the size distribution and composition of the
particles, and must generally be calculated using Mie theory
(assuming equivalent spherical particles). The total light
intensity (direct plus diffuse) depends on both the scattering
and absorption optical depths (τs and τa, respectively). The
maximum light intensity possible is Ix = I0 exp(-τa). The
optical depth can be written as, τ = SML, where S is the
specific cross-section (m2 per gram of particulate), M is the
suspended particle mass concentration (g/m2), and L is the path
length (m). Fine dust and smoke have a Ss of s 1-5 m2/g at
visible wavelengths. However, Sa is very sensitive to the
imaginary index of refrction. For typical soil particles, Sa <
0.1 m2/g. For smokes, Sa can vary from ~ 0.1 to 10 m2/g, roughly
in proportion to the fraction of elemental carbon (graphite) in
the particles. Occasionally, specific extinction coefficients
for smoke are stated relative to the mass of fuel burned; then S
implicitly includes a multiplicative emission factor (grams of
smoke generated per gram of fuel burned).
51. Turco, R. P., 0. B. Toon, R. C. Whitten and P. Hamill,
Trans. Am. Geophys. Union (EOS). 63, 901 (1982).
52. Ogren, J. A., in Particulate Carbon: Atmospheric Life Cycle,
G. T. Wolff and R. L, Klimisch, Eds., Plenum Press, NY, pp.
379-391 (1982).
53. Landsberg, H. E., and J. M. Albert, Weatherwise, 27, 63
(1974).
54. Stommel, H., and E. Stommel, Sci. Amer., 240, 176 (1979).
55. Toon, 0. B., and J. B. Pollack, Natural History, 86, 8
(1977).
56. Lamb, H. H., Climate Present, Past and Future, Vol. 1, Fundamentals and Climate Now, 613 pp.; Vol. 2, Climatic History
and the Future, 835 pp., Methuen, London (1977).
57. Notwithstanding possible alterations in the surface albedo
due to the fires and deposition of soot (see Refs. 15, 74).
58. Milne, D. H., and C. P. McKay, Geological Soc. Amer. Special
Paper 190, L. T. Silver and P. H. Schultz, eds., p. 297 (1982).
59. Toon, 0. B., Trans. Am. Geophys. Union (EOS), 63, 901
(1982).
60. The stratosphere is normally defined as the region of
constant or increasing temperature with increasing height lying
just above the troposphere. The residence time of fine particles
in the stratosphere is considerably longer than in the upper
troposphere due to the greater stability of the stratospheric
air layers and the absence of precipitation in the stratosphere.
With large smoke injections, however the ambient temperature
profile would be substantially distorted and a "stratosphere"
might form in the vicinity of the smoke cloud," increasing its
residence time at all altitudes (Ref. 15). Thus, the timescales
for sunlight attenuation and temperature perturbations in
Figures 1-7 may be considerably underestimated.
61. Transport of soot from the boundary layer into the overlying
free troposphere can occur by diurnal expansion and contraction
of the boundary layer, by large-scale advection, and by strong
localized convection.
62. Barnaby, F., and J. Rotblatt, Ambio, 11, 84 (1982).
63. The term "intermediate" fallout distinguishes the
radioactivity deposited between several days and % 1 month after
an exchange from "prompt" fallout (< 1 day) and "late" fallout
(months to years). The intermediate fallout is expected to be at
least hemispherical in scale, and can still deliver a
significant chronic whole-body gamma ray dose. The intermediate
fallout may also contribute a substantial internal dose, for
example from 131i. The intermediate gamma ray dose represents,
in one sense, the minimum average post-exchange exposure.
However, the geographical distribution of intermediate fallout
would still be highly variable, and estimates of the average
dose made with a one-dimensional model are greatly idealized.
The present calculations were calibrated against the observed
prompt fallout of nuclear test explosions (Ref. 15).
64. There is also reason to believe that the fission yield
fraction of nuclear devices may be increasing as warhead yields
decrease and uranium processing technology improves. If the
fission fraction were unity, our dose estimates would have to be
doubled. This also neglects additional potential sources of
radioactive fallout from salted "dirty" weapons and explosions
over nuclear reactors.
65. Lee, H., and W. E. Strope, Stanford Res. Inst. Report EGU
2981, Menlo Park, CA (1974). The authors studied exposure in the
USA to transoceanic fallout generated by several assumed Si
no-Soviet nuclear exchanges. Taking into account the weathering
of fallout debris, protection by shelters, and a five-day delay
before initial exposure, potential whole-body gamma ray doses 10
rad and internal doses > 10-100 rad, mainly to the thyroid and
intestines, were estimated.
66. These estimates assume normal rates and patterns of
precipitation, which control the intermediate radioactive
fallout. In severely perturbed cases, however, it may happen
that the initial dispersal of the airborne radioactivity is
accelerated by heating, but that intermediate deposition is
suppressed by lack of precipitation over land.
67. Johnston, J., G. Whitten and J. Birks, J. Geophys. Res., 78,
6107 (1973); Johnston, H. S., J. Geophys. Res., 82, 3119 (1977).
68. Gerstl, S. A. W., A. Zardecki and H. L. Wiser, Nature, 294,
352 (1981).
69. Esposito, M. P., T. 0. Tiernan and F. E. Dryden,
Environmental Protection Agency Report EPA-600/280-197 (1980).
70. Josephson, J., Env. Sci. Techno!., _L7_, 124A (1983). In the
burning of PCB's, for example, the release of toxic polycyclic
chlorinated organic compounds can amount to 0.1% by weight. In
the U.S. alone, more than 300,000 tons of PCB's are currently in
use in electrical systems (S. Miller, Env. Sci. Techno!., 17,
11A, 1983).
71. Chen, C.-S., and H. D, Orville (J. Appl. Meteorol., 16, 401,
1977) model the effects of fine graphitic dust on cumulus-scale
convection. Among other findings, they show that strong
convective motions can be established in still air within ten
minutes after the injection of a km-sized cloud of submicron
particles of carbon black, at mixing ratios < 50 parts per
billion by mass. When excess humidity is added in their model to
induce rainfall, stronger convection results; the carbon dust is
raised higher and spread farther horizontally, while < 20% is
scavenged by the precipitation. Gray, W. M., W. M. Frank, M. L.
Torrin and T. A. Stokes (J. Appl. Meteorol., 15, 355, 1976)
discuss possible weather modifications on the mesoscale (> 100
K) due to large carbon dust injections.
72. C. Covey, S. Thompson and S-. Schneider (prvt. comm.) of
NCAR have carried out preliminary GCM simulations using soot
burdens similar to those in our baseline model. They find major
global circulation perturbations within a week of injection.
73. Eagan, R. C, P. V. Hobbs and L. F. Radke, J. Appl. Meteorol.,
U, 553 (1974).
74. Sagan, C, 0. B. Toon, and J. B. Pollack (Science, 206, 1363,
1979) discuss the impact of anthropogenic albedo changes in
global climate. Nuclear war may cause albedo changes in several
ways: by burning large areas of forest and grassland; by
generating massive quantities of soot which can settle out on
plants, snow fields and ocean surface waters; and by altering
the pattern and extent of ambient water clouds. The nuclear
fires in the present model consume an area ~ 1.5 x 106
km2, or only = 1% of the global landmass; it is
doubtful that an albedo variation over such a limited area is
significant. All of the soot in the baseline nuclear war model,
if spread uniformly over the Earth, would amount to a layer ~
0.5 µm thick. Even if the soot settled out uniformly on all
surfaces, the first rainfall would wash it into soils and
watersheds. The question of the effect of soot on snow and ice
fields is currently under debate (J. Birks, prvt. comm., 1983).
In general, soot or sand accelerates the melting of snow and
ice. Soot which settles into the oceans would be rapidly removed
by nonselective filter-feeding plankton, if they survived the
initial darkness. In the present calculations, chemical changes
in stratospheric O3 and NO2 concentrations
cause a very small temperature perturbation compared to that
caused by nuclear dust and smoke; it seems unlikely that
chemically-induced climatic disturbances would be a major factor
in a nuclear war. Tropospheric ozone concentrations, if tripled
(Ref. 7), would lead to a small greenhouse warming of the
surface (W. C. Wang, Y. L. Yung, A. A. Lacis, T. Mo and J. E.
Hansen, Science, 194, 685, 1976). This might result in a more
rapid surface temperature recovery. However, the tropospheric O3
increase is transient (~ 3 months duration) and probably of
secondary importance to the contemporaneous smoke and dust
perturbations.
76. Haberle, R. M., C. B. Leovy and J. B. Pollack, Icarus, 50,
322 (1983).
77. During the Martian dust storm of 1971/71, the IRIS
experiment on Mariner 9 observed that particulates heated the
atmosphere, and produced a vertical temperature gradient that
was substantially subadiabaic (Hanel, R. B., et al., Icarus, 17,
4-23, 1972; Pollack, J. B., et al., J. Geophys. Res., 84, 2929,
1979).
78. A. V. Alexandrov, prvt. comm. (1983); S. Schneider, prvt.
comm. (1983).
79. Thomas, G. E., B. M. Jakosky, R. A. West and R. W. Sanders,
submitted to Geophys. Res. Lett., 1983; B. M. Jakosky, prvt.
comm., 1983.
80. We gratefully acknowledge helpful discussions with Drs. J.
Berry, H. Bethe, C. Dillings, H. Brode, R. Cicerone, L. Colin,
P. Crutzen, R. Decker, P. Dolan, P. Dyal, F. Dyson, P. Ehrlich,
B. Feld, R. L. Garwin, F. Gilmore, L. Grinspoon, A. Kuhl, P.
Morrison, E. Patterson, R. Perret, G. Rawson, J. Rotblatt, E. E.
E. Salpeter, S. H. Schneider, S. Soter and R. Whitten on a
variety of subjects related to this work. We particularly thank
Janet Moore Toll as for compiling information on world
urbanization and May Liu for her assistance with computer
programming.
|