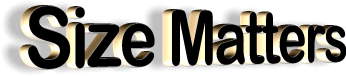
Dr. Hildegarde Staninger
March-29-2007
from
Rense Website
- TABLE 1
- Size of Various
Viruses and Microorganisms as Compared to the Diameter of a Human Hair
-
Note: 10 nanometers (nm) is 1,000 times smaller than
the diameter of the human hair.
-
There are as many nanometers in an inch
as there are inches in 400 miles.
-
-
Category |
Nucleic Acid |
Approx. Size (nm) |
Member Virus |
Natural Hosts |
Papova-virus |
DNA |
45 |
Polyoma |
Mouse |
Adeno-virus |
DNA |
80 |
Types 3, 7, 12, 18 & 31 |
Human |
Retro-viruses |
RNA |
70 to 110 |
Rous sarcoma |
Chicken |
Herpes-virus |
DNA |
100 |
Epstein-Barr |
Human |
Orthomyxoviridae |
RNA |
80-120 |
Influenza A |
Humans, Birds |
|
|
|
|
Mammals |
|
|
|
Influenza B |
Humans, Seals |
|
|
|
Influenza C |
Humans, Pigs |
-
Special Note: The Orthomyxovirdiae are a family of RNA
viruses which, so far as is known, infect mainly vertebrates (Thogotovirus
in ticks, Isavirus in the sea louse). It includes those viruses which cause
influenza.
-
-
Influenza A virus particle or virion is 80-120 nm in
diameter and usually rightly spherical although filamentous forms can occur. A virion has been made by man and is a virus that has
been broken up into 150 pieces.
-
-
Reference:
http://trishul.sci.gu.edu.au/courses/ss12bmi/viruse.html
-
-
-
Origin of Viruses
-
- Viruses are the living dead and they are not cells. There
are three possibilities on the origin of viruses:
-
- If they are
not cells and require living cells for replication, then viruses must not
have been present prior to cellular evolution and could therefore have
coevolved with cells.
-
- Were once
cells but have lost all cellular functions retaining only information to
replicate themselves using hosts machinery.
-
- Viruses have
evolved from plasmids (plasmids are self replicating independent DNA) or
from RNA viroids. These "early" viruses did not contain genes
for capsids. As viroids moved from cell to another, it picked up such genes.
-
- There are some 4000 viruses belonging to 71 families,
11 subfamilies, and 175 genera are known in 1995.
-
- General Characteristics
-
- Viruses (poisons)
are obligate microorganisms, filterable and infectious particles. For example
plant sap with a virus in it can infect other hosts.
-
- 20 nm to
14,000 nm in size.
-
a. Most are smaller than bacteria (eg. Adeno-virus)
-
b. Vaccinia virus is the same size as the small bacteria
like Mycoplasma Rickettsia and Chlamydia.
-
- Contain either
DNA or RNA (never both) surrounded by a protein coat (capsid) and sometimes
covered by an envelope.
-
- Obligate intracellular
entity and multiply inside living cells by using cellular Synthesis machinery
-
-
- TABLE 2
- Comparative Sizes of Biological Objects Diameter
-
-
Limit of Resolution |
-
Biologic Object
|
Micrometer
(um) |
-
Nanometer (nm)
|
-
Human eye (100 um)
|
Ostrich egg |
200,000 |
-
200,000,000
|
|
Mature human ovum |
120 |
-
120,000
|
-
Light microscope (0.2 um)
|
-
Erythrocyte (red blood cell)
|
-
7.5
|
-
7,500
|
|
-
Serratia marcescens
|
-
0.75
|
-
750
|
|
-
Rickettsia species
|
-
0.475
|
-
475
|
|
Clamydia psittaci |
0.27 |
-
270
|
Electron microscope (0.001 um*) |
Mycoplasma species |
0.15 |
-
150
|
|
Influenza virus |
0.085 |
85
|
|
Genetic unit (Muller's Est. of largest size of gene) |
0.02 x 0.1251 |
20 x 1254 |
|
Poliomyelitis virus |
0.027 |
27 |
|
Tobacco necrosis |
plant 0.016 |
16 Virus |
|
Egg albumin molecule (protein molecule) |
0.0025 X 0.01+ |
2.5 x 10+ |
|
Hydrogen molecule |
0.0001 |
0.1 |
-
* For most biologic specimens the limit of resolution
of the electron microscope is 0.001 um, or 1 nm. Under special conditions,
resolution may be obtained at 2 or 3 Angstroms. +Width
x Length
-
-
Taken from: Smith, Alice L. Principles of Microbiology,
10)th (ed). Times Mirror/Mosby College Publishers. St. Louis, Missouri. ©
1995 pg. 145
-
-
- TABLE 3
- Equivalents in the Metric System
-
-
-
Meter
|
-
Centimeter
|
-
Millimeter
|
-
Micrometer8
|
Nanometer8 |
0.1 NM |
Meter (m) 1 |
100 |
1000 |
-
1,000,000
|
1,000,000,000 |
1010 |
Centimeter (cm) |
0.01 |
1 10 |
-
10,000
|
-
10,000,000
|
108 |
-
Millimeter (mm)
|
-
0.001
|
-
0.1
|
1 1000 |
-
1,000,000
|
107 |
-
Micron (u)
|
-
0.000001
|
-
10-4
|
-
10-3 1
|
1000 |
10,000 |
-
Millimicron (mu)
|
-
10-9
|
-
10-7
|
-
10-6
|
10-3 |
101 |
Angstom (A) |
-
0.0000000001
|
|
-
0.0001
|
-
0.1
|
1 |
|
Hydrogen molecule |
0.0001 |
0.1 |
|
|
-
-
Taken from: Smith, Alice L. Principles of Microbiology,
10th (ed).
-
Times Mirror/Mosby College Publishing. St. Louis, Missouri.
© 1995 pg. 144
-
-
-
-
-
-
Excerpts
-
The Surprising Toxicology Of Nanoparticles
-
Size Matters
-
by Trudy E. Bell
-
National Nanotechnology Initiative (NNI)
-
Classification, Definitions, Properties, Hazards,
Risks and Toxicology of Nanoparticles and Nanotechnology
- Size may have another crucial biological consequence:
where nanoparticles end up in the body.
-
- A complex of physical factors such as aerodynamics, gravity,
and mass causes the largest inhalable dust particles to deposit primarily
in the nose and throat. Any toxic effects occur at that site (for example,
nasal cancers due to wood dust). Smaller particles are deposited in upper
airways and are expelled by the "mucosociliary escalator;" the
fingerlike cilia and the mucous lining of the trachea and bronchial tubes,
which together move particles up into the throat and nose, where they are
coughed, sneezed, blown out, or swallowed.
-
-
Any toxic effects usually result
from absorption through the gut (lead poisoning for example).
-
- The next smallest particles penetrate deeper into the
alveolar region (where oxygen and carbon dioxide are exchanged in and out
of the blood) and are usually cleared when alveolar macrophages (special
monocytic scavenger cells in the lungs) engulf the particles and carry
them away.
-
-
But if a high concentration of NSPs (Nano
Scale Particles) is inhaled, the sheer number
of particles - especially if they do not agglomerate - can overwhelm those
clearance mechanisms, and they can penetrate to different parts of the
respiratory tract. Toxic effects are usually due to killing of the macrophages,
which causes chronic inflammation that damages lung tissue (asbestosis
and silicosis are examples).
-
- At sizes less than 100 nanometers, inhaled particles
begin to behave more like gas molecules and can be deposited anywhere in
the respiratory tract by diffusion. Like gases, NSPs - whether natural, incidental,
or engineered - simply because of their "nanoscopic" size, can
pass through the lungs into the bloodstream and to be taken up by cells,
within hours reaching potentially sensitive sites such as bone marrow,
liver, kidneys, spleen, and heart.
-
- As particles become small compared to the size of a cell,
they can begin to interact with the molecular machinery of the cell. The
central nervous system's olfactory bulb (where aromatic molecules are detected)
seems to be able to absorb NSPs smaller than 10 nm from the nasal cavity
- which then can travel along axons and dendrites to cross the blood-brain
barrier.
-
- Inhalation is not the only route into the body. When
ingested, NSPs can end up in the liver, the spleen, and the kidneys. When
touched, NSPs in the range of 50 nm and smaller tend to penetrate the skin
more easily than larger particles (although other aspects such as charge
and surface coatings of the particles are also important), sometimes, being
taken up by the lymphatic system and localizing in the lymph nodes. (See
Figure 3, below.)
-
- By the same token, the mucosociliary escalator is also
not the only way out of the body. There is evidence suggesting that nanoparticles
could be excreted through urine. However, excretion routes for nanoparticles
(urine, feces, sweat) are likely to vary depending on exposure route, size,
charge, surface coating, chemical composition, and many other factors.
-
- For incidental exposure, all this uptake of NSPs into
internal organs could be of concern. But for therapeutic exposure, it is
exciting, as it suggests that engineered nanomaterials can be used to target
therapies to specific organs, even ones normally quite difficult to reach
(such as the brain).
-
- So far, results from different investigators are more
suggestive than definitive. More research needs to be done on methods of
administration, means of uptake, and on the body's clearance mechanisms.
Also, when nanometer-sized particles are generated in combustion processes,
most collide with other particles, are held together by the strong surface
tension, and agglomerate into larger particles.
-
-
The distribution of particles
sizes will depend on the density of nanometer particles at the point of
generation. One of the early priorities for nanotechnology health research
is to gain a better understanding of the particle sizes that are likely
to be associated with the production of engineered nanoparticles.
-
- Still, size is not the only thing that matters for potential
toxicity.
-
- Nanoscale particles can end up in different parts of
the body depending on size and other characteristics as well as routes
of entry. Although many uptake and translocation routes have been demonstrated,
others still are hypothetical and need to be investigated. Translocation
rates are largely unknown, as are accumulation and retention in critical
target sites and their underlying mechanisms. These, as well as potential
adverse effects, largely depend on physicochemical characteristics of the
surface and core of NSPs.
-
-
Both qualitative and quantitative changes in NSP biokinetics in a diseased or compromised organism also need to be considered.
-
-
- Shape Matters
- Although the shapes of NSPs also give them unique properties,
under the Toxic Substances Control Act (TCSA) engineered nanoparticles
may not be viewed as new compounds unless they have a unique composition.
For example, TiO2 nanoparticles are handled the same way with respect to
regulation as bulk TiO2, even though the two forms have different properties.
-
- Some studies show that the materials having the same
composition but of different shapes as well as sizes have different toxicities
- moreover, not with a linear relationship as one might expect. For example,
one study showed that nanoparticles 50 to 130 nm across of quartz-crystalline
silica (a substance known to be toxic) were less toxic than 1.6-mm particles
- but that 10-nm particles were actually more toxic.
-
-
But route of entry
into the body as well as dose also affect toxicity.
-
-
- Purity Matters
-
Bulk carbon in macroscopic components is medically useful
because it is not poisonous to or rejected by the body. Yet, some researchers
have observed from experiments that
carbon nanotubes (especially single-walled
or multi-walled carbon nanotubes) seem to be more toxic than other forms
of carbon. Others have debated that claim because the nanotubes used had
trace impurities of iron or solvents. Indeed, some studies suggest that
other forms of nanoscale carbon such as C60 fullerenes might prevent toxicity
by being antioxidants.
-
- Possibly at stake here, or in similar debates over other
engineered nanomaterials, may be the purity of the engineered nanomaterials.
At this stage, people don't have absolutely repeatable control on manufacturing
processes; nanotech production is now roughly where the production of indium
gallium arsenide phosphide (InGaAsP) semiconductor lasers were in the early
to mid 1980s - relatively low yield of reliable production.
-
-
Thus, buckyball
products from one supplier are not necessarily identical to those from
another, so toxicity may differ.
-
-
Ask sources careful questions about the
size of particles, their manufacture, experimental methods, whether they
characterized the materials themselves at the time when they performed
the experiment or simply believed the statements made by the supplier,
and the comparison of their results with other studies.
-
-
-
-
With more research under way, there are more and new
publications reporting on nanotoxicology.
-
-
Until more is certain, the National
Institute for Occupational Safety and Health (NIOSH) has announced research
needs and interim guidelines for protecting workers in nanotech industries
in its report
Approaches to Safe Nanotechnology.
|